Radially-patterned cell behaviours during tube budding from an epithelium
Preprint posted on 26 April 2018 https://www.biorxiv.org/content/early/2018/04/26/308957
Article now published in eLife at http://dx.doi.org/10.7554/elife.35717
Diving in: a 2D to 3D transition during epithelial tube formation involves not just apical constriction of the invaginating cells but also radially oriented rearrangements of the surrounding cells guided by planar polarized apical myosin II.
Selected by Natalie DyeBackground:
How cellular activity is coordinated in space and time to form properly shaped tissues during development remains an important open question in biology. During tissue morphogenesis, cells can undergo oriented divisions, shape/size changes, neighbour exchanges, or even death; the spatial pattern of each of these morphogenetic behaviours dictates tissue shape. Previous work in 2D epithelial sheets revealed that these cellular activities can be coordinated by cell signalling downstream of patterned gene expression, as well as tissue-scale mechanical stresses. The authors of this preprint extend our knowledge by exploring the cellular dynamics occurring just prior to and during a 2D-3D transition.
Summary of the preprint:
The authors use the Drosophila salivary gland as a model for epithelial tube formation. This organ develops in the embryo from an epithelial placode that invaginates to form a tube in the absence of cell division or death. It was known that a dynamic and pulsatile apical myosin II pool drives apical constriction in the invaginating “pit”, which lies off-center in the placode. By applying and extending new methods for analysing the cellular basis of this transition, the authors of this preprint reveal an additional morphogenetic role for the cells outside the invaginating pit (Fig 1).
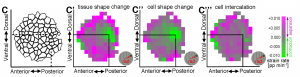
- By tracking apical surfaces, they found that cells far from the pit do not apically constrict but instead undergo oriented rearrangements in a radial pattern: preferentially removing circumferential bonds and growing new radial bonds to expand the tissue toward the pit.
- They correlated the apical surface dynamics with those at a mid-basal position and developed a new method to measure changes in depth.
Fig 2 (Fig 4 in the preprint): diagrams of the 3D cell behaviours involved in tube formation.
- Cells near the pit first contract their apical surface isotropically and then expand the basal side, so that the cells adapt a “wedge” shape (Fig 2).
- Cells away from the pit also become wedge-shaped, but only along the circumferential axis. Along the radial axis, they do not wedge but instead tilt in toward the pit.
- In both regions, rearrangements at the apical and mid-basal positions occur at the same time with similar rates. Nevertheless, the cells far from the pit end up tilted toward one another circumferentially and away from one another radially.
- In cells far from the pit, the authors observe that junctional myosin II is enriched on circumferential bonds (those that will shrink, Fig 3). Mutating forkhead (a specifier of salivary gland) disrupts this tissue-wide organization of myosin II and abolishes pit invagination. While cells remain dynamic in this case, their rearrangements no longer resolve with a radial bias, suggesting that the presence of the invaginating pit and/or the polarized myosin II pattern defines the directionality of the cell rearrangements.
Fig 3 (Fig 6A of the preprint): Tissue-wide patterns of myosin II in the epithelial placode.
What I find interesting:
The quantitative methods used here to span the cellular and tissue length scales are similar, at least in spirit, to those that I use in my own postdoc research (in the group of Suzanne Eaton and Frank Julicher). Thus I am, of course, a fan of the approach and not surprised to see how it provides new mechanistic insight for tissue morphogenesis. A couple points that I find particularly interesting:
- Cell rearrangements can be important – even if they’re noisy.
- Germband extension of the Drosophila embryo is a classic example of convergent extension induced by orienting cell neighbor exchanges. Here, however, the contribution of rearrangements to tissue deformation is harder to appreciate without the quantitative analysis, perhaps in part because of the radial geometry. Many events occur both radially and circumferentially – but the net sum is slightly biased toward extending the tissue radially. Thus, we can think of it perhaps like sailing upwind – zig-zaging back and forth, with indirect progress.
- To explain the mechanism for these rearrangements, the authors make an analogy to germband extension, positing that polarized apical myosin II initiates constriction and a mechanical pulling force from the invaginating pit orients the resolution. I’m not yet fully convinced. Future work to measure the forces and more precisely block apical constriction in the pit, coupled with physical modelling would be really interesting.
- Question: how do the dynamics here compare quantitatively to those during germband extension? Do you see more back and forth neighbour exchanges during salivary gland invagination and if so, what could be the reason for this difference?

- Same players, new game. Just as during germband extension, the cell dynamics observed here correlate with complementing planar polarity patterns for myosin II and the polarity protein Par3/Bazooka. The geometry of the pattern here is radial, whereas in the embryo it is Anterior-Posterior.
- An important future direction will be determine the links between the very upstream fate-specifying transcription factor, Forkhead, and the circumferential pattern of myosin II outside the pit.
- Furthermore, it remains unclear how these two behaviours – apical constriction near the pit and radial rearrangements far from it – are spatially restricted.
- Questions:
- Does the expression of Fog – a GPCR required to enrich myosin II for apical constriction – define these regional domains?
- Could you perturb Fog expression/function to see how the loss of apical constriction affects the directionality of rearrangements and myosin II patterns in the surrounding cells?
- Does the myosin II pattern result from patterned gene expression, mechano-sensitivity, or both?
- The apical side is running the show. Because actin and myosin are largely apically enriched during epithelial morphogenesis (salivary gland and elsewhere), much attention has been paid to this layer. Here, we actually have a very nice quantitative correlation between what’s happening at two different depths during a 2D to 3D transition. The results are consistent with the apical side driving the dynamics and causing the 3D tissue deformation. Nonetheless, it is not immediately obvious how dynamics happening in plane robustly affect out-of-plane 3D tissue deformations. This will be an exciting future direction, and the model system used here seems to be an appropriate simplified tissue for studying this topic.
For more information…
Previous work on salivary gland morphogenesis:
Booth AJR, Blanchard GB, Adams RJ, Röper K. A dynamic microtubule cytoskeleton directs medial actomyosin function during tube formation. Dev Cell. 2014 Jun 9;29(5):562-576. doi: 10.1016/j.devcel.2014.03.023.
Girdler GC, Röper K. Controlling cell shape changes during salivary gland tube formation in Drosophila. Semin Cell Dev Biol. 2014 Jul;31:74-81. doi: 10.1016/j.semcdb.2014.03.020.
Review of Drosophila germband extension:
Kong D, Wolf F, Großhans J. Forces directing germ-band extension in Drosophila embryos. Mech Dev. 2017 Apr;144(Pt A):11-22. doi: 10.1016/j.mod.2016.12.001.
Review of the cellular basis of tissue deformation:
Jülicher F, Eaton S. Emergence of tissue shape changes from collective cell behaviours. Semin Cell Dev Biol. 2017 Jul;67:103-112. doi: 10.1016/j.semcdb.2017.04.004.
Methods for analyzing the cellular basis of tissue deformation:
Blanchard GB. Taking the strain: quantifying the contributions of all cell behaviours to changes in epithelial shape. Philos Trans R Soc Lond B Biol Sci. 2017 May 19;372(1720). pii: 20150513. doi: 10.1098/rstb.2015.0513.
Etournay, R., et. al. Interplay of cell dynamics and epithelial tension during morphogenesis of the Drosophila pupal wing. Elife. 2015 Jun 23;4:e07090. doi: 10.7554/eLife.07090.
Guirao B, et. al. Unified quantitative characterization of epithelial tissue development. Elife. 2015 Dec 12;4. pii: e08519. doi: 10.7554/eLife.08519.
Posted on: 29 May 2018 , updated on: 19 June 2018
Read preprint