Circularly permuted LOV domain as an engineering module for optogenetic tools
Posted on: 1 July 2021 , updated on: 13 July 2021
Preprint posted on 19 May 2021
Article now published in Chemical Communications at http://dx.doi.org/10.1039/d1cc02643g
The circle of LOV: Circularly permuted LOV domain enables photo-control of proteins that require a free N-terminus and expands the applicability of optogenetic tools
Selected by Soni MohapatraCategories: synthetic biology
Background
Optogenetics tools are increasingly being used for the on-demand spatiotemporal control of various cellular processes using light. The light sensitive LOV (light-oxygen-voltage) domain is one of the most commonly used protein domains for modulating a variety of proteins (1). The LOV protein consists of a core, called the PAS (Per-Arnt-Sim) domain and a C-terminal helix, named Jα-helix. Normally, the protein of interest is fused to the Jα-helix of the LOV domain. In the dark state, Jα-helix is stacked against the protein core which cages the protein of interest via stearic hindrance. Upon blue light irradiation, the Jα-helix unfolds which exposes the protein of interest and restores its function (2). Currently, the usage of LOV domain is limited to caging the N-terminus of the proteins and involves optimizing linker lengths between the LOV domain and the protein of interest. Having a more versatile version of the LOV domain can expand the current optogenetic toolkit.
Circular permutation (3) is a method that is adopted to engineer proteins with different connectivity between the amino acids while retaining the three-dimensional structure of the protein. In this preprint, the authors used the circular permutation strategy to re-engineer a version of LOV domain with an accessible C-terminus that can be fused to a wide variety of proteins.
Key findings
Design of a circularly permuted LOV domain
Geng et al. developed a circularly permuted version of LOV (cpLOV) while retaining the light sensing capability of the LOV domain. hLOV1 (4), a version of LOV with a better caging propensity in the dark state was chosen as the candidate for circular permutation. cpLOV was generated by permuting hLOV1 using a glycine-serine linker. hLOV1 was split between L250 and H251 and a new terminus was introduced at the original helix region connecting the Jα-helix and the PAS core. The GSGS linker is sufficiently long to serve as a connector between the original N- and C-terminus of the LOV domain.
cpLOV as a modular domain for controlling protein activity
To test the usability of cpLOV as a caging domain, a hexapeptide SsrA (5) was fused to cpLOV. The choice of the cpLOV fusion site to ssrA has a significant effect on the photo-sensing ability of the SsrA-cpLOV fusion construct. The authors tested ten different fusion sites along the Jα-helix. Yeast surface display was used to evaluate the binding ability of these different SsrA-cpLOV fusion construct to SspB with and without light exposure. The four fusion constructs that displayed light-induced SspB binding were fused away from the central region of the Jα-helix. While the best cpLOV fusion constructs showed better binding ability under light conditions compared to hLOV1, they also displayed undesirable higher background activity in the dark.
The authors also employed cpLOV in a multi-component system to achieve photo-activated transcription in HEK293 cells. They were able to achieve nearly six-fold enhancement of transcriptional activation upon exposure to light.
Improving the peptide caging efficiency of LOV domain using cpLOV
One of the major drawbacks that several photo-controlled proteins suffer from is the lack of sufficient contrast between the light and dark states of these proteins. In this work, hLOV1 is partnered with the engineered cpLOV to act as a dual protein cage and provide tighter caging in the absence of light. As a prototype, tobacco etch virus protease cleavage site (TEVcs) is shielded through a dual cage of hLOV1 and cpLOV1. This doubly caged TEVcs is integrated into a highly sensitive transcriptional assay. In dark conditions, TEVcs is caged, inaccessible to the protease and therefore cannot be cleaved. Upon photo-stimulation and availability of dopamine, TEVcs is uncaged and cleaved by the protease which leads to the downstream expression of the reporter mCherry gene. Geng et al. note a 90% reduction in background activity and demonstrated that dual caging is an effective approach to minimize undesirable background activities of photosensitive proteins.
Why I like this preprint
I am interested in designing photo-controlled helicases and have employed LOV domain as a means to introduce structural modulations to proteins in a light-based manner. This preprint as well as a recently published article by He et al. (6) have introduced circularly permuted versions of LOV that improves the functionality of LOV as a photo-sensing domain. These new circularly permuted versions of LOV can be especially useful for proteins that require a free N-terminus to function. I would be interested in inserting my protein of interest between the PAS domain and the Jα-helix of cpLOV and explore that aspect of cpLOV. The dual caging module that combines hLOV1 and cpLOV to improve the contrast between the light and dark states of the photo-controlled proteins addresses one of the limitations of various optogenetics tools.
Questions for the authors
- How does the lifetime of light/dark states of cpLOV compare to that of hLOV1?
- For the dual caging, a combination of cpLOV and AsLOV2 was used to simultaneously cage the N- and C- terminus of the protein of interest. Is it possible to use two domains of cpLOV instead to achieve similar levels of dual caging effects?
References
- Losi et al. (2018). Blue-light receptors for optogenetics. Chemical Reviews 118,
- Halavaty et al. (2007). N- and C-Terminal Flanking Regions Modulate Light-Induced Signal Transduction in the LOV2 Domain of the Blue Light Sensor Phototropin 1 from Avena sativa. Biochemistry 46, 49.
- Baird et al. (1999). Circular permutation and receptor insertion within green fluorescent proteins. PNAS 96, 11241
- Kim et al. (2017). Time gated detection of protein-protein interactions with transcriptional readout. eLife 6, e30233.
- Song et al. (2003). Structural basis of degradation signal recognition by SspB, a specificity-enhancing factor for the ClpXP proteolytic machine. Molecular cell 12,1.
- He et al. (2021). Circularly permuted LOV2 as a modular photoswitch for optogenetic engineering. Nature Chemical Biology
doi: https://doi.org/10.1242/prelights.29870
Read preprintSign up to customise the site to your preferences and to receive alerts
Register hereAlso in the synthetic biology category:
Enhancer cooperativity can compensate for loss of activity over large genomic distances
Milan Antonovic
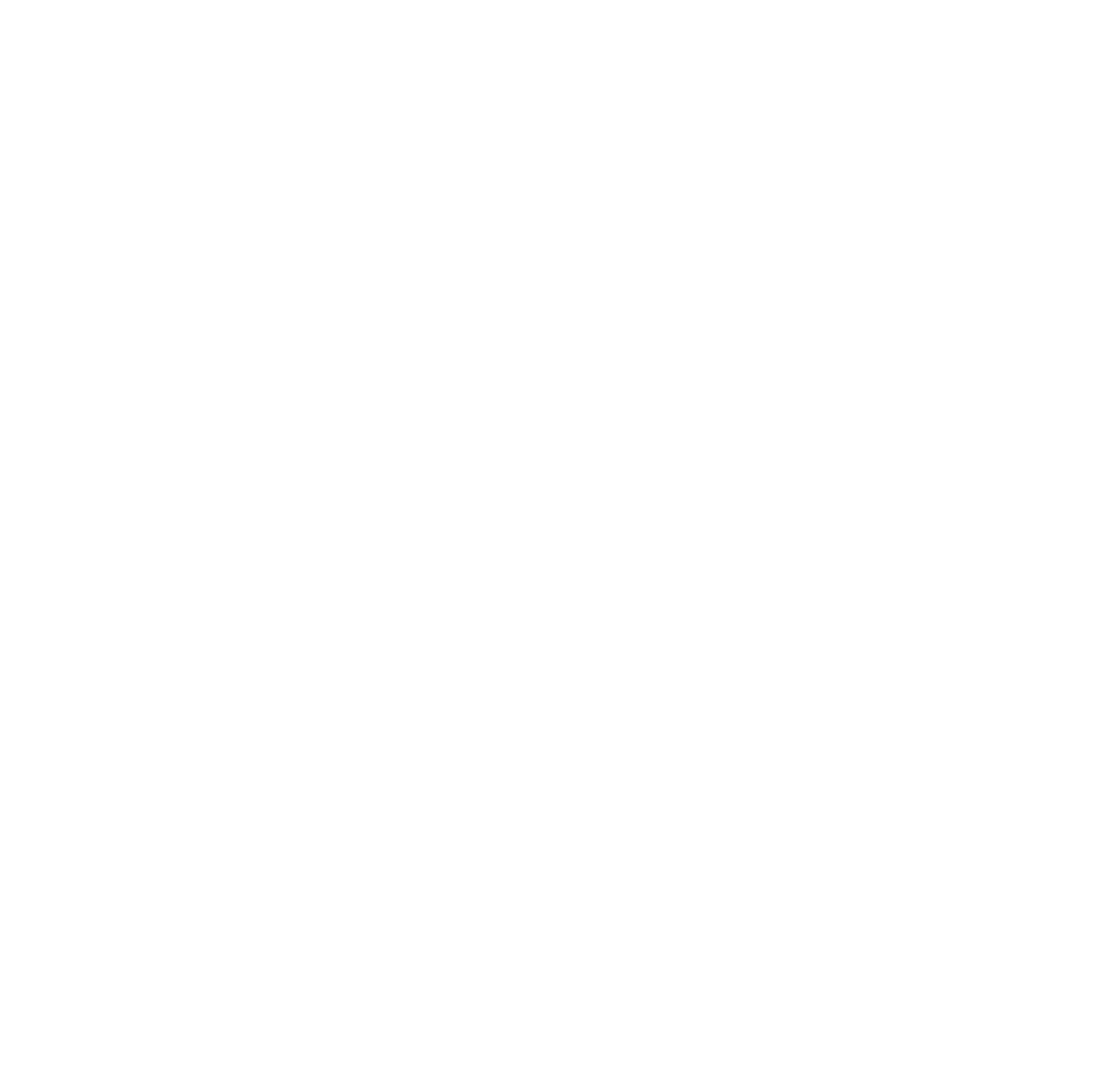
Discovery and Validation of Context-Dependent Synthetic Mammalian Promoters
Jessica L. Teo

Genetically encoded multimeric tags for intracellular protein localisation in cryo-EM
Martyna Kosno-Vega
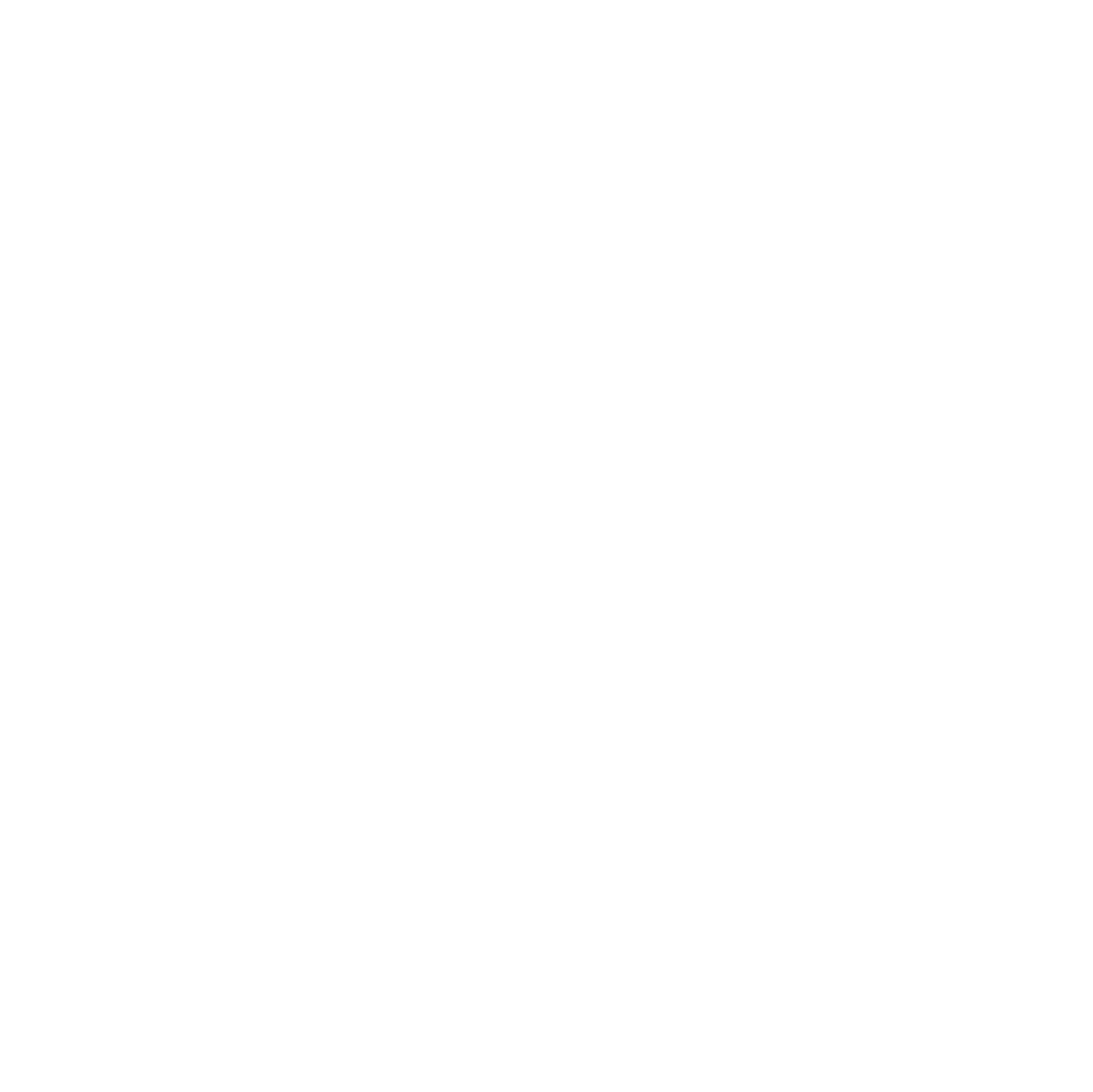
preListssynthetic biology category:
in the‘In preprints’ from Development 2022-2023
A list of the preprints featured in Development's 'In preprints' articles between 2022-2023
List by | Alex Eve, Katherine Brown |
EMBL Synthetic Morphogenesis: From Gene Circuits to Tissue Architecture (2021)
A list of preprints mentioned at the #EESmorphoG virtual meeting in 2021.
List by | Alex Eve |
EMBL Conference: From functional genomics to systems biology
Preprints presented at the virtual EMBL conference "from functional genomics and systems biology", 16-19 November 2020
List by | Jesus Victorino |
Antimicrobials: Discovery, clinical use, and development of resistance
Preprints that describe the discovery of new antimicrobials and any improvements made regarding their clinical use. Includes preprints that detail the factors affecting antimicrobial selection and the development of antimicrobial resistance.
List by | Zhang-He Goh |
Advances in Drug Delivery
Advances in formulation technology or targeted delivery methods that describe or develop the distribution of small molecules or large macromolecules to specific parts of the body.
List by | Zhang-He Goh |