Higher Temperatures Generically Favor Slower-Growing Bacterial Species in Multispecies Communities
Posted on: 26 July 2019
Preprint posted on 2 July 2019
Article now published in Nature Ecology & Evolution at http://dx.doi.org/10.1038/s41559-020-1126-5
Increasing temperature will favor bacteria with slower growth rates, possibly leading them to take over their faster-growing competitors
Selected by Defne SurujonCategories: microbiology
Background
Microbial communities are composed of multiple microbial species that interact with one another and with their common habitat. Such interactions determine the composition, diversity and function of the microbial ecosystem. Environmental perturbations, such as a change in the chemical composition, pH, temperature, or nutrient availability can drastically alter a microbial community. In a complex, multi-species ecosystem, a seemingly simple perturbation may have potentially surprising outcomes. Therefore, predictions on how a change in the environment affects the community composition, diversity and interactions is an exciting area of research.
Recently, researchers from the same lab that published this preprint presented a theoretical model that can predict the outcomes of community composition, in response to changes in the mortality rate (Abreu et al., 2019). The authors show that in a community, slow-growing species are predicted to perform better when mortality is low; and fast-growing species will dominate (become the only remaining species) when mortality is high (Abreu et al., 2019). At intermediate mortality rates, it is possible to have either coexistence of two or more species, or a bistable regime (where either species may dominate).
In this preprint, Lax et al. investigate the influence of temperature. Many microbial communities are subject to changes in temperature through seasonal fluctuations and global warming. However, most microbial studies are conducted at a single, fixed temperature, leaving the effect of such an important factor on community composition and function unexplored. Lax et al. addressed this gap by modeling and experimentally validating community outcomes at different temperatures.
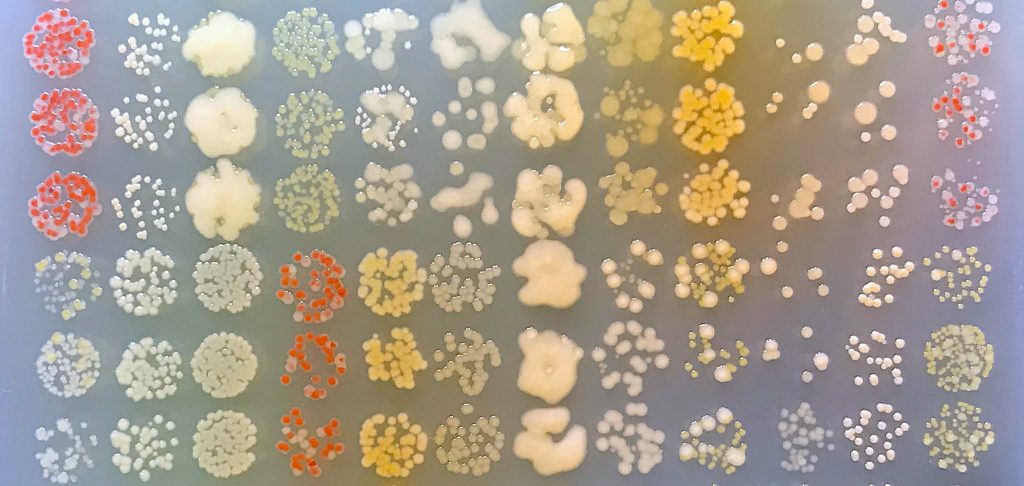
Key Findings
The authors first consider a simple community of two naturally co-occurring bacteria, with different growth rates. For instance, when cultured separately at 16°C, the fast-growing species is 1.3x faster than the slow-grower. When cultured together, the fast-grower dominates, which is quite intuitive. However, at 25°C, the two species coexist, and at 30°C, the slow-grower dominates. This seems especially puzzling, since at increasing temperatures, the fast-grower is a lot faster (2x) than the slow-grower.
The authors then develop a theoretical model, based on their previous work, that can reconcile this counter-intuitive finding. They use a modified version of the competitive Lotka-Volterra model that captures interspecies interactions. In a multi-species community, the growth of a given species depends on several factors:
- The maximal growth rate of the species. Different bacterial species can have drastically different replication times, even at near-optimal growth conditions.
- Inhibition from self. Growth cannot continue indefinitely; there will come a point where a population is larger than the environment can accommodate (i.e. exceeded the carrying capacity).
- Inhibition from other species. Microbes can compete for the same resources, for instance, by secreting antimicrobial toxins to inhibit another species’ growth.
- A community-wide death rate. This is the mortality imparted by the environment, and not by any of the community members. E.g. the physical removal of part of the community in dynamic sites such as the human gut.
How does temperature affect these parameters? The authors use the Ratkowsky model, where, the square root of the growth rate increases linearly with increasing temperature. Lax et al. show that this is indeed the case for 13 species spanning a range of growth rates.
When the authors cultured 38 different pairs coming from the 13 species, they observed that in general, higher temperatures favor the slower grower relative to colder temperatures. Most excitingly, the predictions can also be applied to more than 2-species communities. The authors show a 3-species community that is composed of a slow-, intermediate- and fast-growing species. Their model predicts that the fastest grower should do best at the lowest temperature, the slowest grower should do best at the highest temperature, and the intermediate grower should likely do best at intermediate temperatures. They validate these predictions with empirical data.
Why I Find this Work Exciting
Abiotic factors such as temperature play a very important role in community structure and dynamics. Changes in temperature can result in the microbes’ production of secondary metabolites, or changing the susceptibility of a microbe to other stressful factors (such as altered pH or antimicrobials). While there is a lot of unexplored nuance in how temperature affects a complex community, the remarkable conclusion of this preprint is that it is possible to accurately predict the community outcomes without prior knowledge of these nuances.
This study, and the preceding work from the Gore lab (Abreu et al., 2019), are great examples of how mathematical modeling can help explain biological phenomena, and how seemingly perplexing observations can inform more accurate models. Starting from a relatively simple competitive model, the authors have made empirical observations that the initial model could not predict. Through the incorporation of the community-wide death rate (prior to this preprint), and the effect of temperature on growth rate (this work), they develop an accurate model that applies to communities composed of very different microbial species. The results on the 3-species community also suggests that complex, real-world microbial communities can be modeled. In addition to understanding the dynamics of existing natural communities, this work may also lead to the informed design of synthetic microbial communities for decontamination and bioremediation efforts.
Future Directions and Questions for the Authors
- It would be very exciting to see if the model presented can apply to communities with more than 3-species, e.g. microbiota found on the human body, fermented foods, the soil
- The authors consider a range of fixed temperatures. It would be interesting to see if cycles in temperature (mimicking seasonal changes for example) would affect microbial communities similarly
- In the model, there is a “universal death rate”, assuming all species have identical death rates. Is the “universal death rate” something that can be fine-tuned? e.g. every species has its own, species-specific death rate?
- This model includes competitive interactions. Such interactions are relevant when the species are at close proximity to each other. In a well-mixed setting this can be achieved. But what about a spatially heterogenous situation? Would the authors include models that take into account spatial composition as well?
- Can the theoretical models developed on microbial communities be applied to macro-scale ecosystems?
References
Abreu CI, Friedman J, Woltz VLA, Gore J. Mortality causes universal changes in microbial community composition. Nature Communications. 2019 May 9;10(1):2120.
doi: https://doi.org/10.1242/prelights.12534
Read preprintSign up to customise the site to your preferences and to receive alerts
Register hereAlso in the microbiology category:
Characterization of natural product inhibitors of quorum sensing in Pseudomonas aeruginosa reveals competitive inhibition of RhlR by ortho-vanillin
UofA IMB565 et al.

Feedback loop regulation between viperin and viral hemorrhagic septicemia virus through competing protein degradation pathways
UofA IMB565 et al.
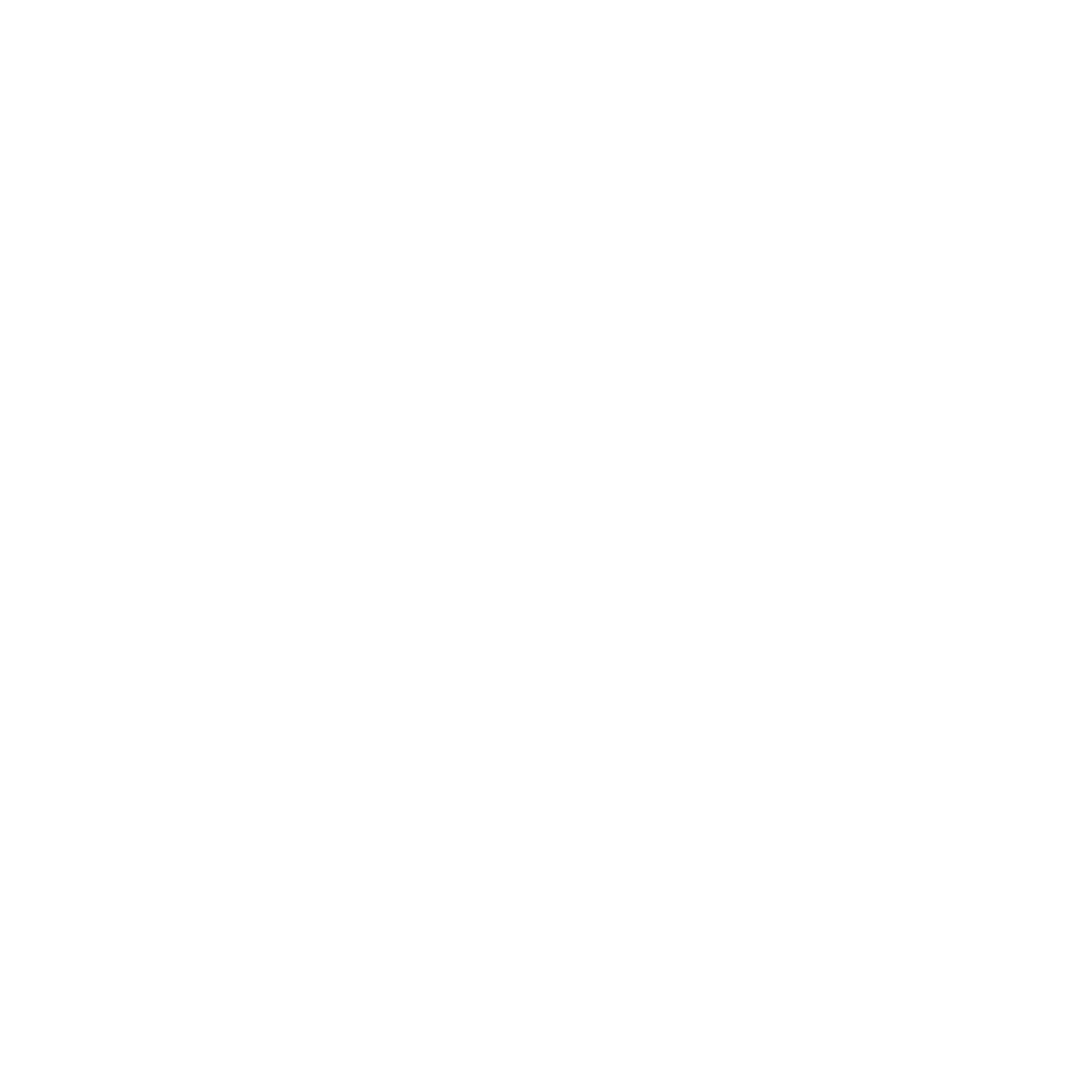
Lytic bacteriophages interact with respiratory epithelial cells and induce the secretion of antiviral and proinflammatory cytokines
UofA IMB565 et al.

preListsmicrobiology category:
in theBioMalPar XVI: Biology and Pathology of the Malaria Parasite
[under construction] Preprints presented at the (fully virtual) EMBL BioMalPar XVI, 17-18 May 2020 #emblmalaria
List by | Dey Lab, Samantha Seah |
1
ECFG15 – Fungal biology
Preprints presented at 15th European Conference on Fungal Genetics 17-20 February 2020 Rome
List by | Hiral Shah |
EMBL Seeing is Believing – Imaging the Molecular Processes of Life
Preprints discussed at the 2019 edition of Seeing is Believing, at EMBL Heidelberg from the 9th-12th October 2019
List by | Dey Lab |
Antimicrobials: Discovery, clinical use, and development of resistance
Preprints that describe the discovery of new antimicrobials and any improvements made regarding their clinical use. Includes preprints that detail the factors affecting antimicrobial selection and the development of antimicrobial resistance.
List by | Zhang-He Goh |