Functional MRI of large scale activity in behaving mice
Posted on: 18 May 2020
Preprint posted on 18 April 2020
Into the brain: Exploring the big picture of complex neural activity in awake mice.
Selected by Mariana De NizCategories: animal behavior and cognition, neuroscience
Background
Adaptive behaviour requires dynamic interactions between neurons and neural circuits across many brain regions. Access to study neuronal circuits in rodents has provided important insights into how local neuronal activity relates to behaviour. While studies focusing on specific regions of the brain at any one time have given us key insights into the function in such regions and the connections involves, a brain-wide view is key to understand how interactions betweenregions gives rise to behaviour, for example, how interactions between the motor cortex, the basal ganglia, the cerebellum, and the thalamus result in motor learning and execution. A brain-wide approach would also help in guiding, in an unbiased way, the selection of brain regions to be studied at higher detail. In their preprint, Fonseca et al (1) present a novel method based on functional magnetic resonance imaging (fMRI), to study interconnections between brain areas to answer outstanding and previously inaccessible behavioural and neurological questions. Moreover, this technique allows for longitudinal and non-invasive studies, and can be used in rodent models and in humans, bridging findings across species.
Key findings and developments
Tool development
The authors used fibre optics, pressure sensors, and 3D printed parts to generate an fMRI setup that includes a head-fixation system, multiple sensory delivery systems for olfactory and visual stimuli (input), and three separate behavioural measures (licking and right and left lever press) (output) (Figure 1).
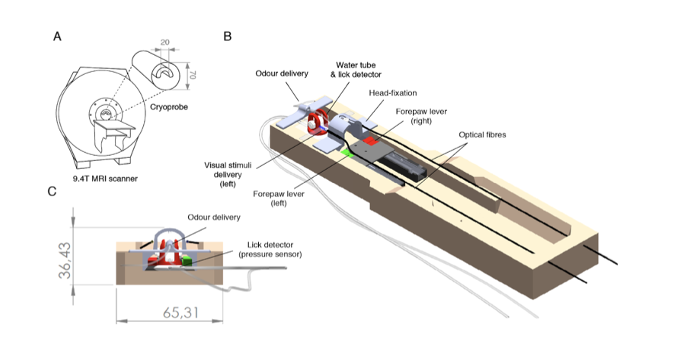
Biological findings
Using this setup, mice were trained in a classical conditioning task, whereby they learned to associate different odours (conditioned stimuli), with biologically relevant (unconditioned stimuli) outcomes, such as the presence or absence of water rewards. This was chosen to allow identifying brain activity as related to odour identity and reward association. An identified challenge, and possibly a confounder, is the stress experienced by mice undergoing MRI. To minimize stress, the authors implemented 4 steps: 1) to train the mice to voluntarily enter the head-fixation apparatus; 2) gradual exposure to MRI sounds only once the mice were proficient in the tasks, as stress is known to influence learning; 3) maintaining sounds at the highest level in the study for at least 7 days to allow for acclimation; 4) habituating the mice to the vibrations of the scanner. While measuring brain-wide activity by recording blood-oxygenation-level-dependent fMRI signals across the brain, the authors detected that despite minimal brain movement, large amplitude changes in brain signals were temporally coupled with lick events -which require jaw and tongue movements. The authors aimed to correct these artefacts, not at the behavioural level, but at the analysis level. They therefore analysed information present in the muscle tissue to predict and remove the artefacts observed in the brain, and found muscle-based pre-processing was effective at correcting the artefacts.
Key findings in this study using the fMRI approach were, first, confirmation that there are distinct spatial activity patterns for different odours in the olfactory bulb –odourant receptors are arrayed into a spatial map of glomeruli, such that each odour activates a unique spatial pattern of activity. Second, the mapping of both motor and reward correlates in the odour guided classical conditioning task. Activity was detected during the odour period, in the primary olfactory (piriform) cortex, while in the outcome period, multiple cortical and subcortical areas were engaged. This included motor-related regions as well as various areas involved in memory , autonomic regulation, and aversion and appetite responses.
Third, in a separate task, they explored the voluntary preparatory action (lever pressing), unlinked to the reflexive action of licking. This offers the possibility to study the readout for cognitive phenomena such as decision-making, and the neural correlates in this process. Press-related brain activity showed sequential activation of the anterior cingulate area, and the primary motor cortex, followed by the secondary motor cortex, the dorsal striatum and the upper limb region of the somatosensory cortex (SSp(UL)). These were finally followed by activity in the ipsilateral SSp(UL).
Fourth, they explored neural correlates of preparatory (lever pressing) and consummatory behaviour (licking). They found that right lever presses preferentially engaged the upper region of the somatosensory cortex in the contralateral hemisphere, while licking correlated best with the mouth region, and was more bilateral. Lever-pressing and licking also recruited various cortical and subcortical regions. Because light was used as stimulus in the task, the visual cortex and the superior colliculus were also engaged.
Decoding approaches further allowed investigating task-related activity. This included comparison of valid and invalid responses and their relation to reward contingencies. The areas identified as discriminating between the two conditions, were regions previously implicated in associative learning, decision-making, motivation, action planning, as well as initiation of consummatory actions, reward processing, and memory. Among their findings, the authors propose for the first time, that the piriform cortex may play a role in non-olfactory associative processes.
Behaviour depends not only on intra-area local processing but critically on the interaction and coordination of information processing across multiple areas. The authors studied functional coupling between brain regions upon performance of the different tasks. Among the interesting findings was the identification of the retrosplenial (RSP) cortex as being a key active area during lever pressing. The RSP is at the intersection of areas that encode visual information, motor feedback, higher-order decision-making, and hippocampal formation. The findings in this work are consistent with suggestions that the RSP may specifically contribute to cognitive processing, by integrating visual and motivational cues, with information generated by self-motion (2).
Altogether, the authors explored fMRI to gain information about functional coupling and activation across brain regions during behaviour. They envisage that this information can be used to define areas to explore using complementary invasive circuit techniques, and propose other possible types of analysis can be performed with fMRI beyond the one explored here, including how functional coupling changes over time, and how it relates to functional connectivity.
What I like about this preprint
I like the rationale of using a technique that allows bridging findings across species – this is explored and discussed as a key point in this paper. Equally, I like work that has in mind the big picture. I think conventionally, research tends to be very specific, and although this of course has led to a lot of discoveries, it has its shortcomings. I see as a positive thing that we are entering a different time, where the interconnectivity of systems (be this systems, circuits, organs, host-pathogen interactions, etc) is seen as equally important to understand. And I think since we started heading in this direction, we have discovered things we couldn’t have imagined before. I like this paper because a) it goes in this direction of exploring a full organ during the performance of specific tasks, and because b) the authors developed the instrument/setup that allows such research. Tool development is what often precedes the questions we can ask, and this will be key for the advances we can achieve.
Open questions
- I think you did a great job addressing the fact that when an animal is stressed, this affects its ability to learn and perform tasks – you addressed this by helping the animals acclimatize gradually to the setup. Is there any way in which in the future, this setup can be further optimized to allow measurements in a free-moving animal?
- Are there studies in humans already, of a similar nature? fMRI has been used in humans already. Do you know if and to what level your findings correlate with the behavior and cognition in the human brain?
- You investigated various complex processes beyond motor activity and reward association, namely, decision-making, motivation, and memory. If you can correlate this to findings in humans, many diseases affecting the central nervous system could be studied in rodent models. Are you interested in any specific ones for which there are rodent models?
- Beyond studies in the brain, fMRI can be used to study for instance, the spinal cord. Do you envisage further developing your method to allow investigating activity beyond the brain? And therefore covering diseases that affect both the central and peripheral nervous system.
- Considering the global picture, what is the main limitation you found in the work you performed (be this the setup, or the findings), and how would you address it/them in the future?
References
- Fonseca MS, Bergomi MG, Mainen ZF, Shmesh N, Functional MRI of large scale activity in behaving mice, bioRxiv, 2020
- Cooper BG, and Mizumori SJY, Temporary inactivation of the retrosplenial cortex causes a transient reorganization of spatial coding in the hippocampus, J Neurosci, 21(11), 2001
doi: https://doi.org/10.1242/prelights.20673
Read preprintSign up to customise the site to your preferences and to receive alerts
Register hereAlso in the animal behavior and cognition category:
Blue appendages and temperature acclimation increase survival during acute heat stress in the upside-down jellyfish, Cassiopea xamachana
Maitri Manjunath

Experience-dependent plasticity of a highly specific olfactory circuit in Drosophila melanogaster
T. W. Schwanitz

Behavioral screening of conserved RNA-binding proteins reveals CEY-1/YBX RNA-binding protein dysfunction leads to impairments in memory and cognition
Chee Kiang Ewe

Also in the neuroscience category:
Sexually dimorphic role of diet and stress on behavior, energy metabolism, and the ventromedial hypothalamus
Jimeng Li

Enhancer-driven cell type comparison reveals similarities between the mammalian and bird pallium
Rodrigo Senovilla-Ganzo

Autism gene variants disrupt enteric neuron migration and cause gastrointestinal dysmotility
Rachel Mckeown

preListsanimal behavior and cognition category:
in the9th International Symposium on the Biology of Vertebrate Sex Determination
This preList contains preprints discussed during the 9th International Symposium on the Biology of Vertebrate Sex Determination. This conference was held in Kona, Hawaii from April 17th to 21st 2023.
List by | Martin Estermann |
Bats
A list of preprints dealing with the ecology, evolution and behavior of bats
List by | Baheerathan Murugavel |
FENS 2020
A collection of preprints presented during the virtual meeting of the Federation of European Neuroscience Societies (FENS) in 2020
List by | Ana Dorrego-Rivas |
Also in the neuroscience category:
‘In preprints’ from Development 2022-2023
A list of the preprints featured in Development's 'In preprints' articles between 2022-2023
List by | Alex Eve, Katherine Brown |
CSHL 87th Symposium: Stem Cells
Preprints mentioned by speakers at the #CSHLsymp23
List by | Alex Eve |
Journal of Cell Science meeting ‘Imaging Cell Dynamics’
This preList highlights the preprints discussed at the JCS meeting 'Imaging Cell Dynamics'. The meeting was held from 14 - 17 May 2023 in Lisbon, Portugal and was organised by Erika Holzbaur, Jennifer Lippincott-Schwartz, Rob Parton and Michael Way.
List by | Helen Zenner |
ASCB EMBO Annual Meeting 2019
A collection of preprints presented at the 2019 ASCB EMBO Meeting in Washington, DC (December 7-11)
List by | Madhuja Samaddar et al. |
SDB 78th Annual Meeting 2019
A curation of the preprints presented at the SDB meeting in Boston, July 26-30 2019. The preList will be updated throughout the duration of the meeting.
List by | Alex Eve |
Autophagy
Preprints on autophagy and lysosomal degradation and its role in neurodegeneration and disease. Includes molecular mechanisms, upstream signalling and regulation as well as studies on pharmaceutical interventions to upregulate the process.
List by | Sandra Malmgren Hill |
Young Embryologist Network Conference 2019
Preprints presented at the Young Embryologist Network 2019 conference, 13 May, The Francis Crick Institute, London
List by | Alex Eve |