DropSynth 2.0: high-fidelity multiplexed gene synthesis in emulsions
Posted on: 23 August 2019 , updated on: 27 August 2019
Preprint posted on 20 August 2019
Article now published in Nucleic Acids Research at http://dx.doi.org/10.1093/nar/gkaa600
Gene Synthesis Costs Reduced to a Drop in the Bucket – DropSynth2.0 Improves Multiplexed Gene Synthesis
Selected by Connor RosenCategories: synthetic biology, systems biology
Background:
In the last two decades, the ability to rapidly sequence DNA with ever-decreasing costs has revolutionized biology, generating vast amounts of genomic data and unlocking new areas of biology. A variety of highly multiplexed assays taking advantage of high-throughput DNA synthesis with next-generation DNA sequencing to interrogate function of DNA variants, generalized as Multiplexed Assays for Variant Effects (MAVEs), have revealed new biological insights at an incredible pace and scale [Gasperini 2016]. However, the ability to generate gene-size (100s-1000s of DNA base pairs) libraries with high fidelity has limited the scale and scope of some functional assays. Previously, the Kosuri group described DropSynth, an inexpensive bead- and emulsion-based method to assemble gene-size DNA fragment from short oligo pools [Plesa and Sidore, 2018]. Here, they improve DropSynth to substantially increase the scale and fidelity, enabling even high-throughput generation of high-quality gene libraries.
Key findings:
A major improvement in DropSynth fidelity (the percentage of assemblies that perfectly match the designed sequence) came from optimization using high-fidelity polymerases, resulting in a ~5-fold improvement to a ~20% rate of perfect assemblies across multiple different codon usage libraries. Several other steps were tested for improved fidelity or to support the use of the high-fidelity polymerases, including buffer optimization, enzymatic mismatch correction, and suppression PCR. Finally, the scale of DropSynth was improved by expanding the on-bead barcode repertoire to enable 4-times as many assembly reactions to be carried out at once. Together, these technical optimizations represent a substantial improvement in the rate and cost of gene synthesis using the DropSynth technique.
Importance:
Gene synthesis can still be a rate- or cost-limiting step in the design and implementation of high-throughput functional assays. DropSynth initially developed a robust low-cost synthesis platform for variant library production, enabling what the authors described in their first manuscript as “broad mutational scanning”. However, the fidelity of assembly was still too low for some applications. DropSynth 2.0 greatly improved the scale and success of this technique, and illustrates the promise for further refinement to enable ever-greater assembly of gene libraries. One exciting area this promises to open is the functional interrogation of the rapidly-expanding gene databases generated by large-scale genome and metagenome sequencing efforts. For example, the ability to rapidly generate libraries of all predicted polyketide synthase and non-ribosomal peptide synthase domains (as just one example) from microbial metagenomes will be important to enable functional interrogation of the full gene content of often uncultured microbes.
Moving Forward / Questions for Authors:
- What and where are the major types of errors in gene synthesis with DropSynth? One might expect that errors will be more enriched at the overlap sequences than in the intervening sequence (where it should be limited to the rate of polymerase error), but the next-generation sequencing data should clarify that assumption. Additionally, what percentage of erroneous sequences have indels that result in early stop codons? If a substantial fraction of errors result in truncated proteins, functional selection (e.g. cloning in-frame with GFP to enable sorting of full-length variants) may be sufficient for downstream applications rather than requiring dial-out PCR or similar techniques when a high fraction of perfect assemblies is required.
- Is DropSynth primarily limited by number of oligos per gene, or by the length of the overall assembly? As private companies increase the length of oligo pools offered, what do the authors expect to be the limiting step in determining the size of genes that may be produced by DropSynth?
- There seem to be substantial differences between the two different codon libraries prepared. Does this reflect differences in assembly fidelity / efficiency, PCR bias during sequencing library preparation, or simply the variability between library assembly attempts?
- The example libraries are of near-uniform size, which enables size selection and efficient bulk suppression PCR. In a situation where many different genes are assembled of varying lengths (such as the domain-scale metagenome libraries described above), how efficient do the authors expect the bulk suppression PCR to be, or what other methods of removing low molecular weight and improperly assembled products might be used?
References:
- Gasperini M., Starita L., Shendure J. “The power of multiplexed functional analysis of genetic variants” 2016. Nature Protocols 11, 1782-1787
- Plesa C.*, Sidore A.M.*, Lubock N.B., Zhang D., Kosuri S. “Multiplexed gene synthesis in emulsions for exploring protein functional landscapes” 2018. Science 359(6373), 343-347
doi: https://doi.org/10.1242/prelights.13560
Read preprintSign up to customise the site to your preferences and to receive alerts
Register hereAlso in the synthetic biology category:
Enhancer cooperativity can compensate for loss of activity over large genomic distances
Milan Antonovic
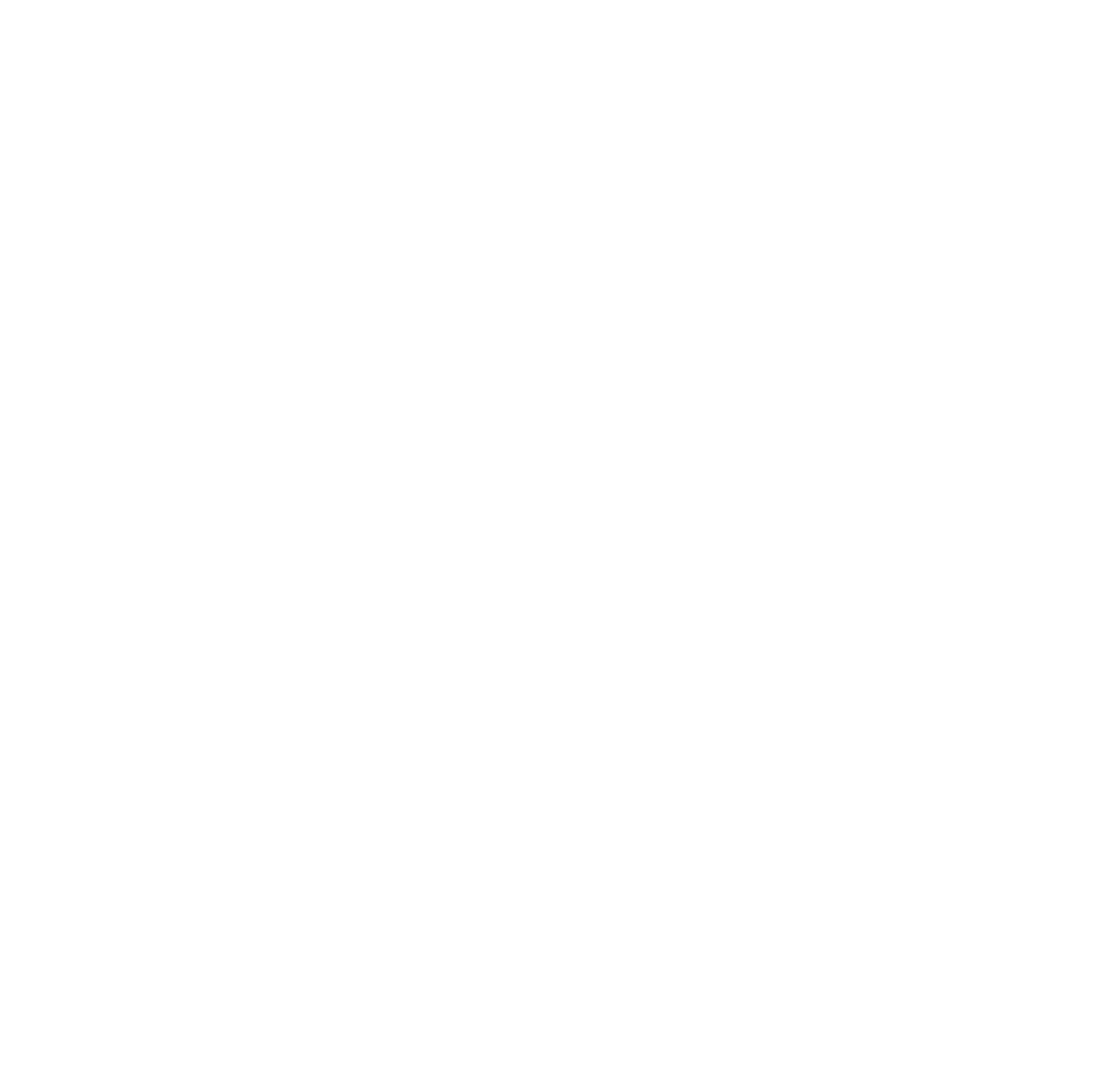
Discovery and Validation of Context-Dependent Synthetic Mammalian Promoters
Jessica L. Teo

Genetically encoded multimeric tags for intracellular protein localisation in cryo-EM
Martyna Kosno-Vega
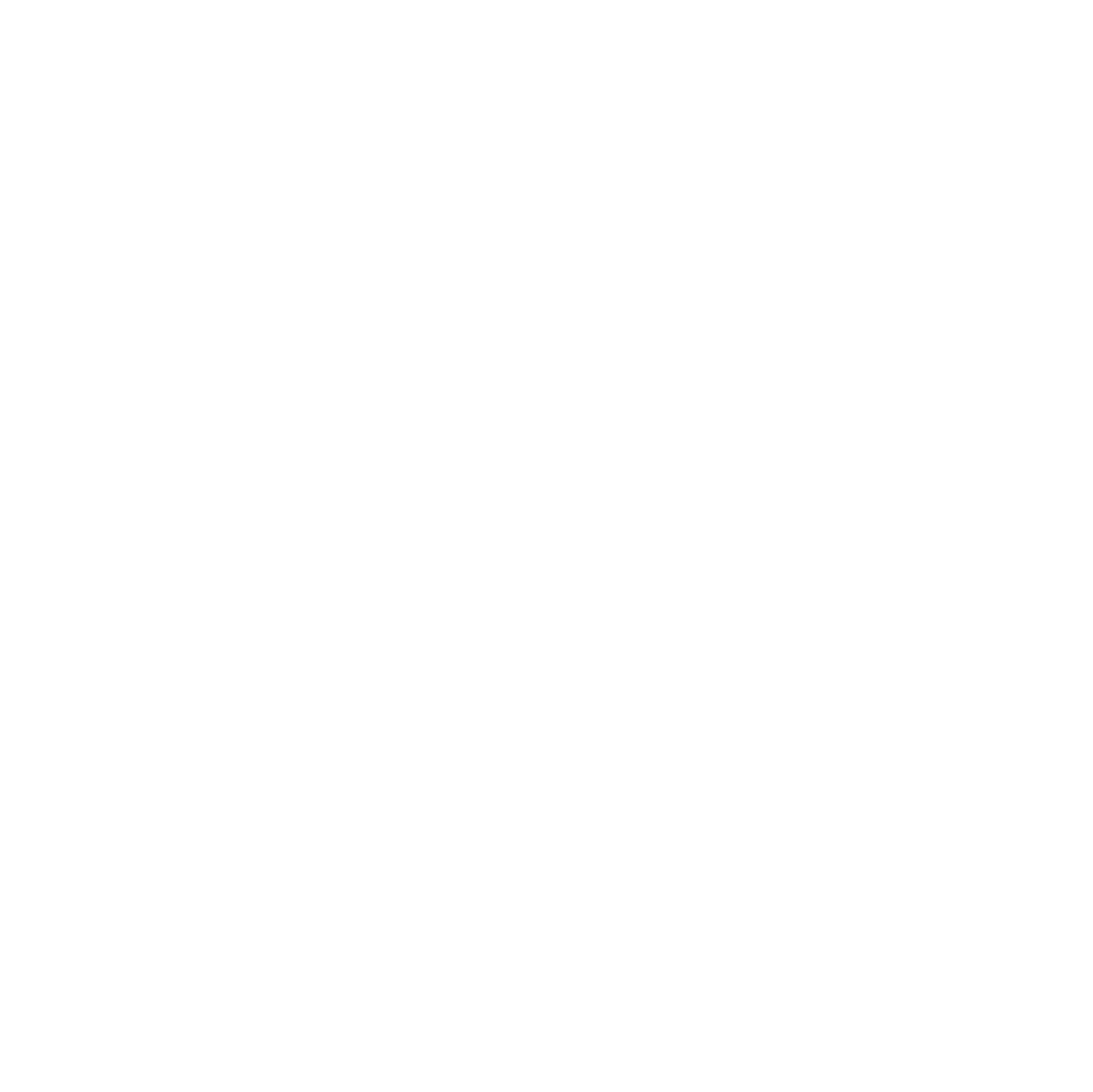
Also in the systems biology category:
Modular control of time and space during vertebrate axis segmentation
AND
Natural genetic variation quantitatively regulates heart rate and dimension
Girish Kale, Jennifer Ann Black
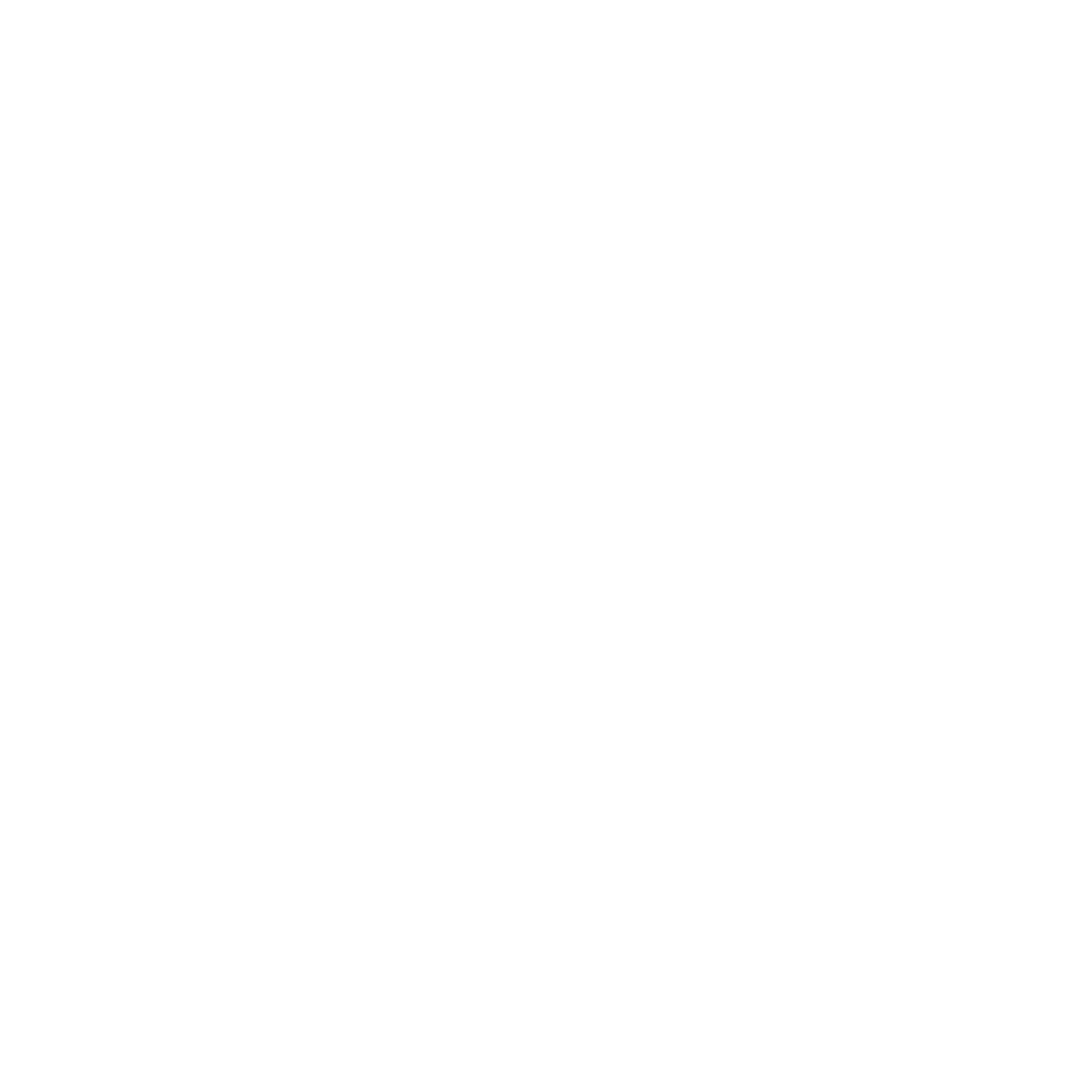
Expressive modeling and fast simulation for dynamic compartments
Benjamin Dominik Maier
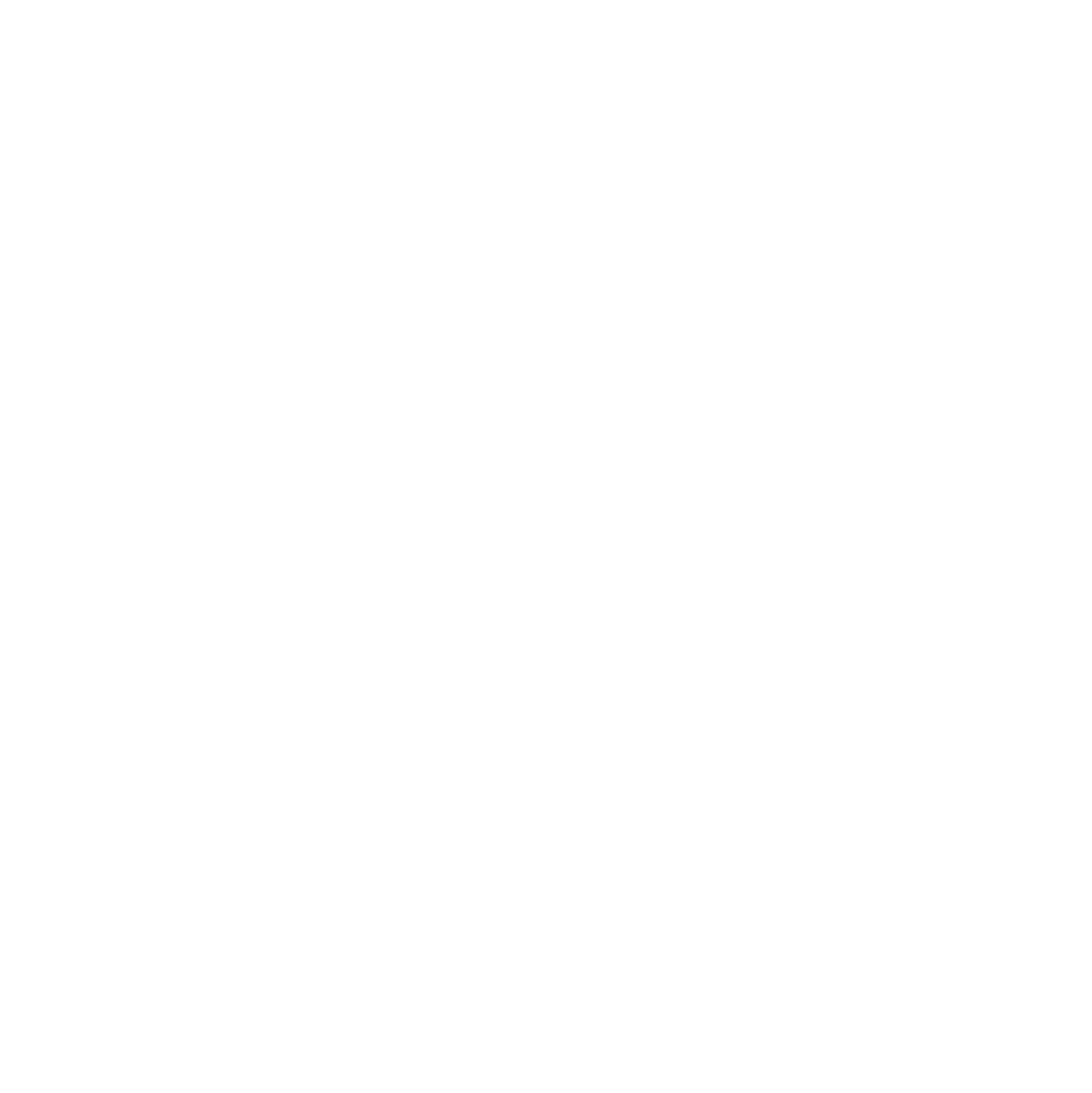
Clusters of lineage-specific genes are anchored by ZNF274 in repressive perinucleolar compartments
Silvia Carvalho

preListssynthetic biology category:
in the‘In preprints’ from Development 2022-2023
A list of the preprints featured in Development's 'In preprints' articles between 2022-2023
List by | Alex Eve, Katherine Brown |
EMBL Synthetic Morphogenesis: From Gene Circuits to Tissue Architecture (2021)
A list of preprints mentioned at the #EESmorphoG virtual meeting in 2021.
List by | Alex Eve |
EMBL Conference: From functional genomics to systems biology
Preprints presented at the virtual EMBL conference "from functional genomics and systems biology", 16-19 November 2020
List by | Jesus Victorino |
Antimicrobials: Discovery, clinical use, and development of resistance
Preprints that describe the discovery of new antimicrobials and any improvements made regarding their clinical use. Includes preprints that detail the factors affecting antimicrobial selection and the development of antimicrobial resistance.
List by | Zhang-He Goh |
Advances in Drug Delivery
Advances in formulation technology or targeted delivery methods that describe or develop the distribution of small molecules or large macromolecules to specific parts of the body.
List by | Zhang-He Goh |
Also in the systems biology category:
2024 Hypothalamus GRC
This 2024 Hypothalamus GRC (Gordon Research Conference) preList offers an overview of cutting-edge research focused on the hypothalamus, a critical brain region involved in regulating homeostasis, behavior, and neuroendocrine functions. The studies included cover a range of topics, including neural circuits, molecular mechanisms, and the role of the hypothalamus in health and disease. This collection highlights some of the latest advances in understanding hypothalamic function, with potential implications for treating disorders such as obesity, stress, and metabolic diseases.
List by | Nathalie Krauth |
‘In preprints’ from Development 2022-2023
A list of the preprints featured in Development's 'In preprints' articles between 2022-2023
List by | Alex Eve, Katherine Brown |
EMBL Synthetic Morphogenesis: From Gene Circuits to Tissue Architecture (2021)
A list of preprints mentioned at the #EESmorphoG virtual meeting in 2021.
List by | Alex Eve |
Single Cell Biology 2020
A list of preprints mentioned at the Wellcome Genome Campus Single Cell Biology 2020 meeting.
List by | Alex Eve |
ASCB EMBO Annual Meeting 2019
A collection of preprints presented at the 2019 ASCB EMBO Meeting in Washington, DC (December 7-11)
List by | Madhuja Samaddar et al. |
EMBL Seeing is Believing – Imaging the Molecular Processes of Life
Preprints discussed at the 2019 edition of Seeing is Believing, at EMBL Heidelberg from the 9th-12th October 2019
List by | Dey Lab |
Pattern formation during development
The aim of this preList is to integrate results about the mechanisms that govern patterning during development, from genes implicated in the processes to theoritical models of pattern formation in nature.
List by | Alexa Sadier |