14-3-3 binding regulates Tau assembly and microtubule association
Posted on: 3 May 2024 , updated on: 9 May 2024
Preprint posted on 15 March 2024
14-3-3 and Tau have a molecular situationship - only specific interactions prevent a tangled protein mess
Selected by Barbora Knotkova, Jannik Faierson, preLights peer supportCategories: biochemistry
written by: Jannik Faierson
Master student in Biochemistry, Heidelberg University, Heidelberg, Germany
Background
A common characteristic of neurodegenerative diseases is the accumulation of protein aggregates in the brain (1). So far, researchers could link individual aggregating proteins to specific diseases. However, the underlying molecular pathogeneses diverge across disorders because small changes in protein size, shape, quantity, and lifetime can already have a significant impact on its interactions (2). Thus, to better understand disease progressions for targeted therapeutic intervention, it is vital to study the individual processes of aggregation. What initiates it? By which mechanism? And is it a symptom or rather a cause?
Tau is an intrinsically disordered protein that associates with microtubules in neurons (3). Due to the absence of extensive secondary structure elements, Tau is highly flexible which makes it very accessible for (hyper-)phosphorylation, leading to dissociation from microtubule (4,5). Although free Tau is abundant and soluble in the healthy brain, it aggregates in Alzheimer’s disease with such a regularity and intensity that it is commonly termed a hallmark (4,6). In previous work, the authors of this preprint demonstrated that Tau undergoes liquid-liquid-phase-separation (LPPS) before forming aggregates (6). However, the underlying molecular mechanism remains elusive.
14-3-3 proteins comprise a large family of regulatory chaperones that recognize phosphorylated target proteins. Isoforms of 14-3-3 proteins are especially ubiquitous in the brain. Their role in neurodegeneration, however, is controversial. Initially, it has been shown that downregulating 14-3-3 is linked to deficits in brain function (7). However, significant amounts of 14-3-3 proteins were also identified in aggregates (8). An important binding partner of 14-3-3ζ is Tau (6). It is unknown whether the chaperone protects it from aggregating, or rather contributes to it. In this ‘intrinsically disordered’ research gap, Hochmair et al. contribute a comprehensive mechanistic model that explains the role of 14-3-3ζ in Tau physiology, supported by an array of biochemical data.
Main findings
- The molar ratio between Tau and 14-3-3ζ determines whether Tau forms condensates.
The most important takeaway is that stoichiometric binding of 14-3-3ζ dimers suppresses Tau aggregation. The authors could show with in vitro fluorescent microscopy imaging that condensation of recombinantly expressed and in vitrophosphorylated Tau (p-Tau) significantly decreased as 14-3-3ζ concentrations increased. When both reached stoichiometric amounts, Tau was fully solubilized. By solving the crystal structure of 14-3-3ζ dimers with a Tau-derived peptide, Hochmair et al. validated previous research (9) showing that phosphorylated serine residues at positions S214 and S324 are required for 14-3-3ζ binding. In the absence of these two phosphorylated serines, or at substochiometric levels of 14-3-3ζ, multivalent electrostatic interactions drove the co-condensation of positively charged Tau domains and the negatively charged 14-3-3ζ. This was especially apparent from results showing that unphosphorylated Tau, which has a lower propensity to phase separate, can be driven into LLPS by the addition of 14-3-3ζ. Finally, out-competing 14-3-3ζ binding of p-Tau with other substrates also enhances condensation and phase separation. In short, only the presence of stoichiometric 14-3-3ζ amounts can shield the p-Tau from electrostatically driven condensation. As soon as the balance tips towards free p-Tau, it phase separates together with 14-3-3ζ.
- Binding of 14-3-3ζ to p-Tau promotes dissociation from microtubules.
The authors found that p-Tau binds 14-3-3ζ with a similar affinity to microtubules (MT). Using in vitro pelleting assays, they demonstrated that adding 14-3-3ζ increases p-Tau dissociation from MTs while not affecting unphosphorylated Tau’s attachment to them. Further experiments involving fluorescent microscopy assays of in vitro polymerized MTs confirmed that, after adding 14-3-3ζ, p-Tau no longer co-localizes with the microtubules. Thus, 14-3-3ζ may directly prevent the accumulation of unbound p-Tau in the cytosol by “scavenging” p-Tau before it dissociates. The resulting reversible ‘handing-over’ of p-Tau from MTs to 14-3-3ζ may explain how soluble p-Tau can exist in high concentrations without aggregating.
- Tau aggregation can be inhibited by 14-3-3ζ in vivo.
Hochmair et al. concluded this study by testing whether the insights they gained also hold true in more complex in vivo models. They expressed a GFP-tagged aggregation-prone mutant of Tau (found in frontotemporal dementia patients) and visualized its distribution in primary hippocampal mouse neurons. Treatment with a 14-3-3ζ binding inhibitor increased Tau aggregation in a dose-dependent manner, supporting the authors’ hypothesis of a 14-3-3ζ ‘buffer system’. Based on this finding, the authors emphasize the need for caution when using unspecific kinase inhibitors to tackle p-Tau aggregation in neurodegeneration: the loss of interaction-promoting serine phosphorylation on Tau might have the same effect as the tested 14-3-3ζ binding inhibitor. Unphosphorylated Tau might not be able to bind 14-3-3ζ and aggregate independently of stoichiometric concentrations.
Conclusion
The researchers have developed a model showing how Tau condensation, aggregation, and microtubule binding are regulated by 14-3-3ζ. It was informed by the discovery of two different modes of interaction: 1) the phosphorylated residues S214 and S324, as supported by the crystal structure, promote specific binding between the two proteins; and 2) unspecific multivalent electrostatic interactions drive 14-3-3ζ-p-Tau co-condensation. In stoichiometric conditions, 14-3-3ζ can “scavenge” p-Tau directly from the MTs and thus prevent accumulation in the cytosol that eventually drives Tau condensation and aggregation. Crucially, if competitive or irreversible inhibitors interfere with this binding, or if there isn’t enough 14-3-3 present, the balance is tipped towards condensation.
What we like about this preprint
Hochmair et al. integrate previous research and their various findings into a model showing how 14-3-3ζ affects Tau condensation. They connect the loose ends by testing hypotheses (such as binding of 14-3-3ζ to Tau increases solubility) with a wide range of biochemical methods: while crystallography elucidates the underlying structure and potential modes of interaction, fluorescent microscopy on in vitro and in vivo model systems shows solubility behavior that is in line with the proposed model outlined above. The latter can be robustly subjected to further testing – by e.g. chemically modifying 14-3-3ζ for increased p-Tau affinity – to elucidate whether interfering with the 14-3-3 chaperone system or designing 14-3-3 mimics can have neuroprotective effects. Ultimately, by understanding the molecular interactions between Tau and 14-3-3, the authors of this preprint provide a potential path for researchers to develop more targeted therapeutical approaches for Alzheimer’s disease.
Questions
How does 14-3-3ζ prevent Tau from aggregating when it only specifically interacts with a small part of Tau, especially given that the termini of Tau are flexible?
It seems like the R127A mutant of 14-3-3ζ was just as good as WT 14-3-3ζ at dissolving condensates of unphosphorylated Tau at stoichiometric ratios, but was worse at dissolving PKA-Tau condensates compared to WT. Do you have an explanation for this?
What could be a plausible source of Tau aggregation in AD patients? Out-competition of 14-3-3ζ by other binding partners, downregulation of 14-3-3, or something else?
Have you tried to express a phosphomimetic mutant of S214 S324 in the primary hippocampal mouse neurons to show that a potential increase in affinity/constitutive binding would actively prevent aggregate formation?
Is 14-3-3ζ upregulated in neural stress situations similar to, for example, heat shock proteins, and if not, can we upregulate it for therapeutics?
References
- Ross CA, Poirier MA. Protein aggregation and neurodegenerative disease. Nat Med. 2004; 10(7): S10. https://doi.org/10.1038/nm1066
- Mahler HC, Friess W, Grauschopf U, Kiese S. Protein aggregation: Pathways, induction factors and analysis. Vol. 98, Journal of Pharmaceutical Sciences. John Wiley and Sons Inc.; 2009. p. 2909–34. https://doi.org/10.1002/jps.21566
- Guo T, Noble W, Hanger DP. Roles of tau protein in health and disease. Vol. 133, Acta Neuropathologica. Springer Verlag; 2017. p. 665–704. https://doi.org/10.1007/s00401-017-1707-9
- Soeda Y, Takashima A. New Insights Into Drug Discovery Targeting Tau Protein. Vol. 13, Frontiers in Molecular Neuroscience. Frontiers Media S.A.; 2020. https://doi.org/10.3389/fnmol.2020.590896
- Liu F, Li B, Tung EJ, Grundke-Iqbal I, Iqbal K, Gong CX. Site-specific effects of tau phosphorylation on its microtubule assembly activity and self-aggregation. Eur J Neurosci. 2007; 26(12): 3429.https://doi.org/10.1111/J.1460-9568.2007.05955.X
- Wegmann S, Biernat J, Mandelkow E. A current view on Tau protein phosphorylation in Alzheimer’s disease. Vol. 69, Current Opinion in Neurobiology. Elsevier Ltd; 2021. p. 131–8. https://doi.org/10.1016/j.conb.2021.03.003
- Wegmann S, Eftekharzadeh B, Tepper K, Zoltowska KM, Bennett RE, Dujardin S, et al. Tau protein liquid–liquid phase separation can initiate tau aggregation. EMBO J. 2018 Apr 3; 37(7). https://doi.org/10.15252/embj.201798049
- Gu Q, Cuevas E, Raymick J, Kanungo J, Sarkar S. Downregulation of 14-3-3 Proteins in Alzheimer’s Disease. Mol Neurobiol. 2020 Jan 1; 57(1): 32–40. https://doi.org/10.1007/s12035-019-01754-y
- McFerrin MB, Chi X, Cutter G, Yacoubian TA. Dysregulation of 14-3-3 proteins in neurodegenerative diseases with Lewy body or Alzheimer pathology. Ann Clin Transl Neurol. 2017 Jul 1; 4(7): 466–77. https://doi.org/10.1002/acn3.421
- Neves JF, Petrvalská O, Bosica F, Cantrelle FX, Merzougui H, O’Mahony G, et al. Phosphorylated full-length Tau interacts with 14-3-3 proteins via two short phosphorylated sequences, each occupying a binding groove of 14-3-3 dimer. FEBS Journal. 2021 Mar 1; 288(6): 1918–34. https://doi.org/10.1111/febs.15574
doi: https://doi.org/10.1242/prelights.37229
Read preprintSign up to customise the site to your preferences and to receive alerts
Register hereAlso in the biochemistry category:
Structural basis of respiratory complexes adaptation to cold temperatures
Pamela Ornelas
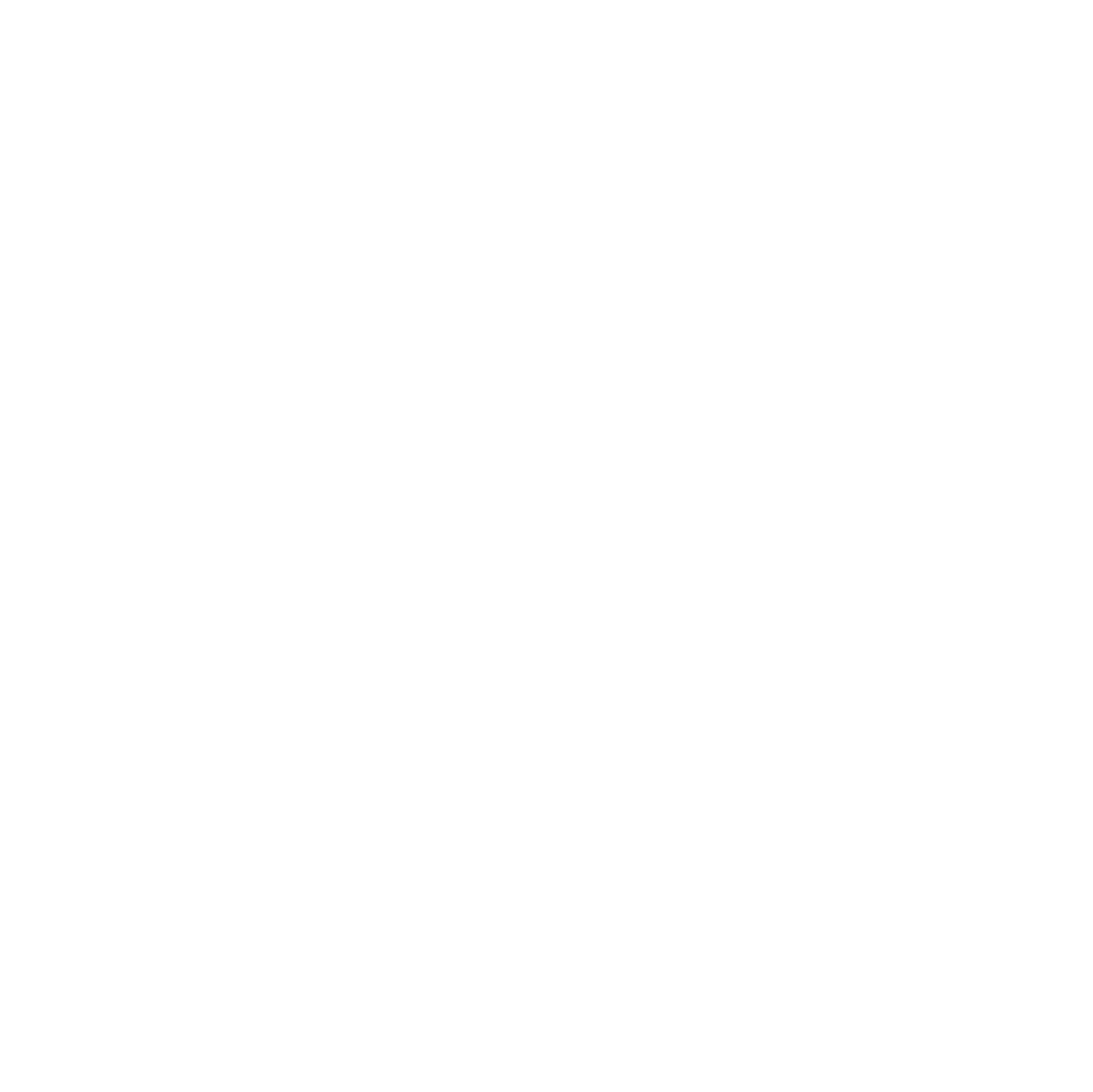
Lens Placode Modulates Extracellular Matrix Formation During Early Eye Development
Bhaval Parmar
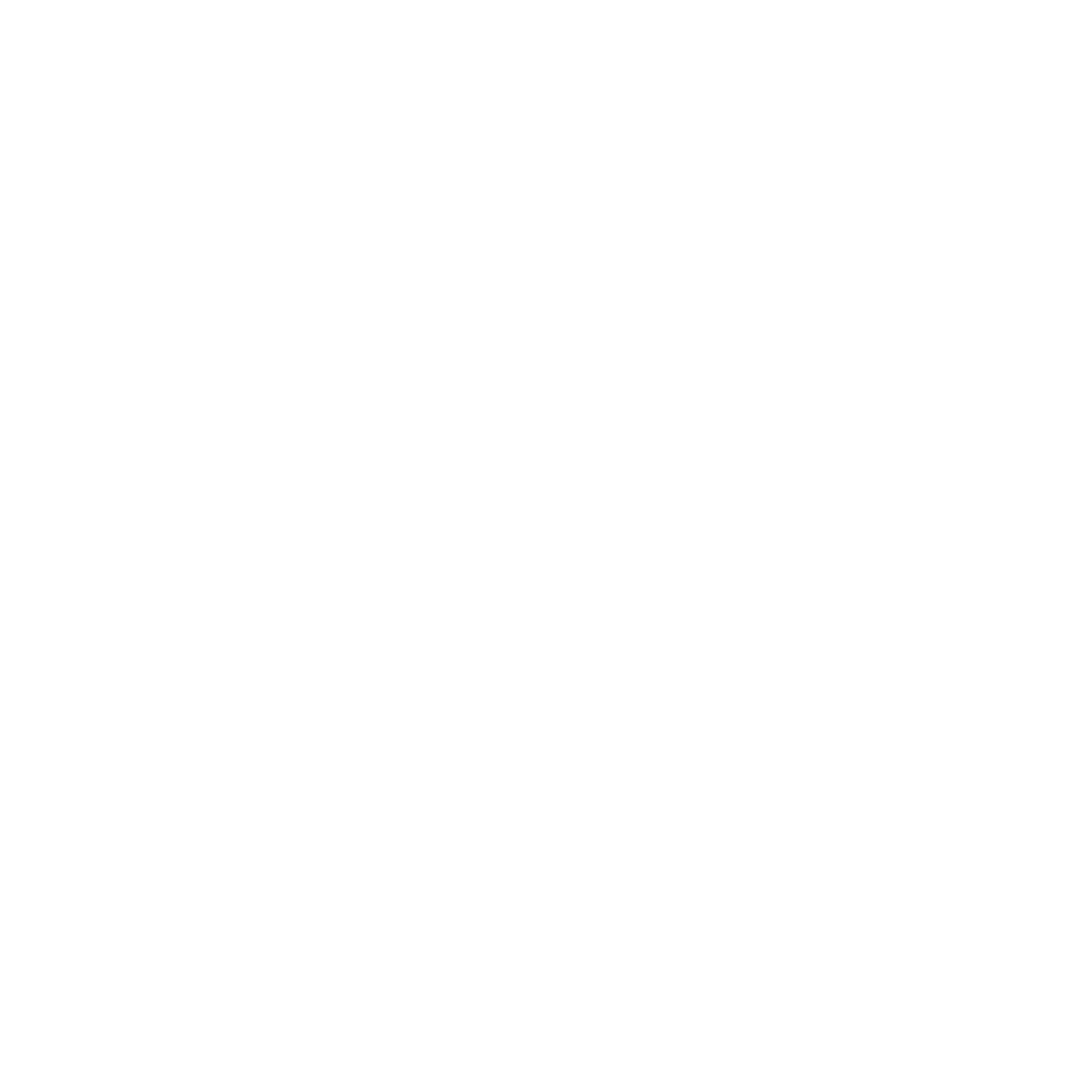
Generalized Biomolecular Modeling and Design with RoseTTAFold All-Atom
Saanjbati Adhikari

preListsbiochemistry category:
in theBSCB-Biochemical Society 2024 Cell Migration meeting
This preList features preprints that were discussed and presented during the BSCB-Biochemical Society 2024 Cell Migration meeting in Birmingham, UK in April 2024. Kindly put together by Sara Morais da Silva, Reviews Editor at Journal of Cell Science.
List by | Reinier Prosee |
Preprint Peer Review – Biochemistry Course at UFRJ, Brazil
Communication of scientific knowledge has changed dramatically in recent decades and the public perception of scientific discoveries depends on the peer review process of articles published in scientific journals. Preprints are key vehicles for the dissemination of scientific discoveries, but they are still not properly recognized by the scientific community since peer review is very limited. On the other hand, peer review is very heterogeneous and a fundamental aspect to improve it is to train young scientists on how to think critically and how to evaluate scientific knowledge in a professional way. Thus, this course aims to: i) train students on how to perform peer review of scientific manuscripts in a professional manner; ii) develop students' critical thinking; iii) contribute to the appreciation of preprints as important vehicles for the dissemination of scientific knowledge without restrictions; iv) contribute to the development of students' curricula, as their opinions will be published and indexed on the preLights platform. The evaluations will be based on qualitative analyses of the oral presentations of preprints in the field of biochemistry deposited in the bioRxiv server, of the critical reports written by the students, as well as of the participation of the students during the preprints discussions.
List by | Marcus Oliveira |
CellBio 2022 – An ASCB/EMBO Meeting
This preLists features preprints that were discussed and presented during the CellBio 2022 meeting in Washington, DC in December 2022.
List by | Nadja Hümpfer et al. |
20th “Genetics Workshops in Hungary”, Szeged (25th, September)
In this annual conference, Hungarian geneticists, biochemists and biotechnologists presented their works. Link: http://group.szbk.u-szeged.hu/minikonf/archive/prg2021.pdf
List by | Nándor Lipták |
Fibroblasts
The advances in fibroblast biology preList explores the recent discoveries and preprints of the fibroblast world. Get ready to immerse yourself with this list created for fibroblasts aficionados and lovers, and beyond. Here, my goal is to include preprints of fibroblast biology, heterogeneity, fate, extracellular matrix, behavior, topography, single-cell atlases, spatial transcriptomics, and their matrix!
List by | Osvaldo Contreras |
ASCB EMBO Annual Meeting 2019
A collection of preprints presented at the 2019 ASCB EMBO Meeting in Washington, DC (December 7-11)
List by | Madhuja Samaddar et al. |
EMBL Seeing is Believing – Imaging the Molecular Processes of Life
Preprints discussed at the 2019 edition of Seeing is Believing, at EMBL Heidelberg from the 9th-12th October 2019
List by | Dey Lab |
Cellular metabolism
A curated list of preprints related to cellular metabolism at Biorxiv by Pablo Ranea Robles from the Prelights community. Special interest on lipid metabolism, peroxisomes and mitochondria.
List by | Pablo Ranea Robles |
MitoList
This list of preprints is focused on work expanding our knowledge on mitochondria in any organism, tissue or cell type, from the normal biology to the pathology.
List by | Sandra Franco Iborra |