Soaring styles of extinct giant birds and pterosaurs
Posted on: 26 November 2020 , updated on: 17 December 2020
Preprint posted on 17 November 2020
Article now published in PNAS Nexus at http://dx.doi.org/10.1093/pnasnexus/pgac023
From flying aces to soar losers: In their new preprint, Goto et al predict that not all giant extinct fliers were equally adept in the air.
Selected by Sophia FriesenCategories: animal behavior and cognition, biophysics, paleontology
Background and context:
When picturing a pterosaur, one of the winged reptiles that lived throughout most of the Mesozoic Era, most people imagine it in majestic flight, soaring high above the ancient landscape. Yet recent research by Goto et al. suggests that one of the largest of these animals, Quetzalcoatlus northropi, spent most of its time on the ground, only flying for short distances in emergencies. Quetzalcoatlus’ 10-meter wingspan was about three times that of the longest-winged living bird, the wandering albatross, but despite this, the researchers have determined that it was a poor flier. However, the smaller pterosaur Pteranodon, as well as two giant extinct birds, Pelagornis sandersi and Argentavis magnificens, were most likely adept at soaring on thermal updrafts.
The researchers came to these conclusions through a physics-based analysis of soaring ability for these four giant extinct fliers. Using a model of “dynamic soaring”, which today’s seabirds use to travel long distances by extracting energy from wind gradients over the ocean, as well as a more comprehensive analysis of “thermal soaring”, in which birds like eagles and vultures ride thermal updrafts, the researchers were able to predict the soaring abilities and required wind conditions for the four extinct fliers.
Key findings:
- Quetzalcoatlus and Argentavis were poor dynamic soarers, and the dynamic soaring ability of Pteranodon and Pelagornis depended on uncertain morphological variables
Dynamic soaring harnesses differences in wind speed at different altitudes over the sea surface. Birds like albatrosses and petrels build up speed in high-velocity winds at higher altitude, then travel in a different direction in slower winds closer to the sea. By cycling between different wind speeds and adjusting their angles of flight, they can even fly upwind without flapping. The authors used a physics-based model of dynamic soaring, previously only applied to albatrosses (1) to predict how fast ancient fliers could fly using dynamic soaring, both upwind and downwind, and what wind conditions they would require.
Quetzalcoatlus and Argentavis required minimum wind speeds that were at least twice as fast as those for living dynamic soarers, and they were slower fliers under most wind conditions. The predicted soaring ability of Pteranodon varied depending on wing drag estimates: when a birdlike drag coefficient was used, Pteranodon’s thermal soaring speeds were predicted to meet or exceed modern seabirds’, although it was limited to a narrower set of wind conditions. However, wind-tunnel tests of reconstructed pterosaur wings have produced higher estimates of wing drag (2), and when the higher drag estimate was used, Pteranodon was predicted to be a poor dynamic soarer overall. Pelagornis’ predicted thermal soaring ability was strongly influenced by estimates of body mass; if it were heavier, it would have been a strong dynamic soarer in fast winds, but if it were lighter, it would be worse at dynamic soaring than living species.
- Except for Quetzalcoatlus, the ancient fliers were excellent thermal soarers
Modern birds such as hawks and condors use thermal soaring, circling in thermal updrafts to gain altitude, then gliding over and down to the next updraft. Thermal soaring ability includes two main aspects: how quickly a flier loses altitude as it glides in a straight line, and how quickly it loses altitude when circling (which can also be thought of as the upward wind speed required to keep the flier from sinking). All of the extinct fliers except for Quetzalcoatlus had circling abilities comparable to modern thermal soaring birds, and the straight-line gliding performance of Argentavis, Pelagornis, and Pteranodon was at least as good as living soarers. In fact, depending on uncertain estimates of wing drag and weight, Pelagornis and Pteranodon may have significantly exceeded modern birds in straight-line gliding ability.
Quetzalcoatlus, on the other hand, was predicted to lose altitude in circling flight much more quickly than the living birds tested, including the kori bustard, a mostly terrestrial bird which flies only rarely. This very poor circle-gliding ability would most likely prohibit Quetzalcoatlus from thermal soaring. Given that this extremely large pterosaur was likely unable to perform either type of soaring, and that its flapping flight would have been quite metabolically costly (3), the authors predict that Quetzalcoatlus spent most of its time on the ground.
Why I liked this paper:
As a non-paleontologist, my primary experience of extinct creatures is through the visually detailed reconstructions that are common in popular media. Especially in the era of CGI, it’s too easy to interact with these nearly photorealistic images as if they were live footage, taking for granted that they are accurate. I enjoyed this paper because it made me reconsider the massive amount of work that goes into a well-considered reconstruction. These creatures only exist on the earth as fossilized bones; everything we know about the way they actually lived is based in the kind of painstaking analysis exemplified by this paper. I also appreciate how the researchers went beyond previous studies in taking into account the physical effects of a wider variety of morphological variables that influence flight. This includes, for instance, how wing length would limit how closely a soaring flier could approach the ocean’s surface, as well as recent estimates of wing drag (2) and body weight (4). Changes in these interrelating variables lead to vastly different predictions of soaring ability.
Questions for the authors:
- It’s surprising to me that we still don’t know the shape of the wind gradient over the ocean. How is this usually measured?
- You mention that the model of dynamic soaring gives unrealistically high maximum soaring speeds for some wind speed values. Is this cause for concern that the model isn’t predictive of real flight performances? Are the wind speeds at which the simulation becomes problematic likely in real-world conditions?
- The more recent estimates of pterosaur body weight are around three times previous ones, which makes a huge difference to flight ability. Why are these newer estimates so dramatically different?
References:
- Sachs G (2005). Minimum shear wind strength required for dynamic soaring of albatrosses. Ibis 147(1): 1-10.
- Palmer C (2011). Flight in slow motion: aerodynamics of the pterosaur wing. Proc R Soc B Biol Sci 278(1713): 1881-1885.
- Witton MP, Habib MB (2010). On the size and flight diversity of giant pterosaurs, the use of birds as pterosaur analogues and comments on pterosaur flightlessness. PLoS One 5(11): e13982.
- Henderson DM (2010). Pterosaur body mass estimates from three-dimensional mathematical slicing. J Vertebrate Paleontol 30(3): 768-785.
doi: https://doi.org/10.1242/prelights.25928
Read preprintSign up to customise the site to your preferences and to receive alerts
Register hereAlso in the animal behavior and cognition category:
Geometric analysis of airway trees shows that lung anatomy evolved to enable explosive ventilation and prevent barotrauma in cetaceans
Sarah Young-Veenstra

A depth map of visual space in the primary visual cortex
Wing Gee Shum, Phoebe Reynolds

Neural Basis of Number Sense in Larval Zebrafish
Muhammed Sinan Malik

Also in the biophysics category:
Motor Clustering Enhances Kinesin-driven Vesicle Transport
Sharvari Pitke
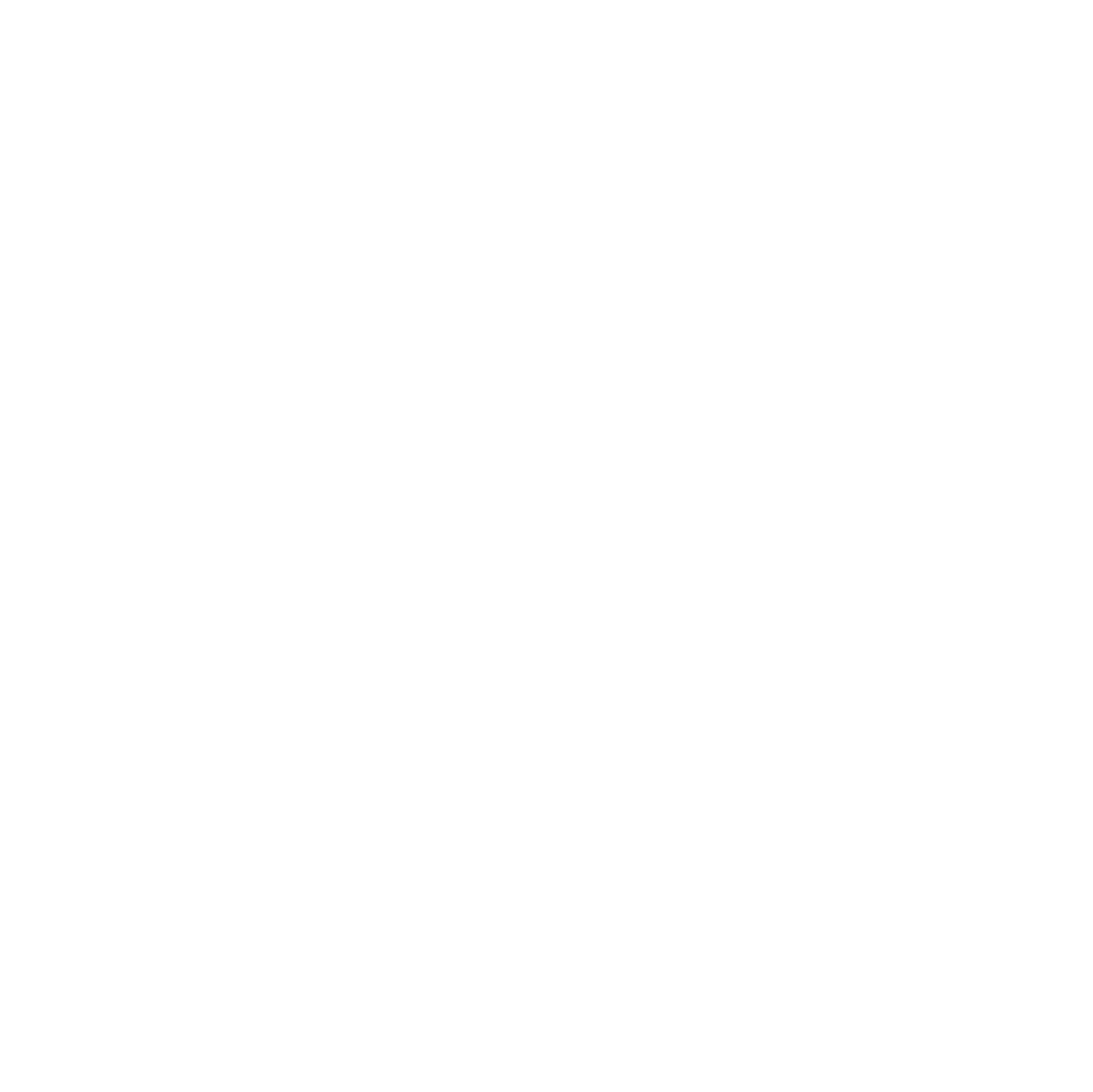
Global coordination of protrusive forces in migrating immune cells
yohalie kalukula
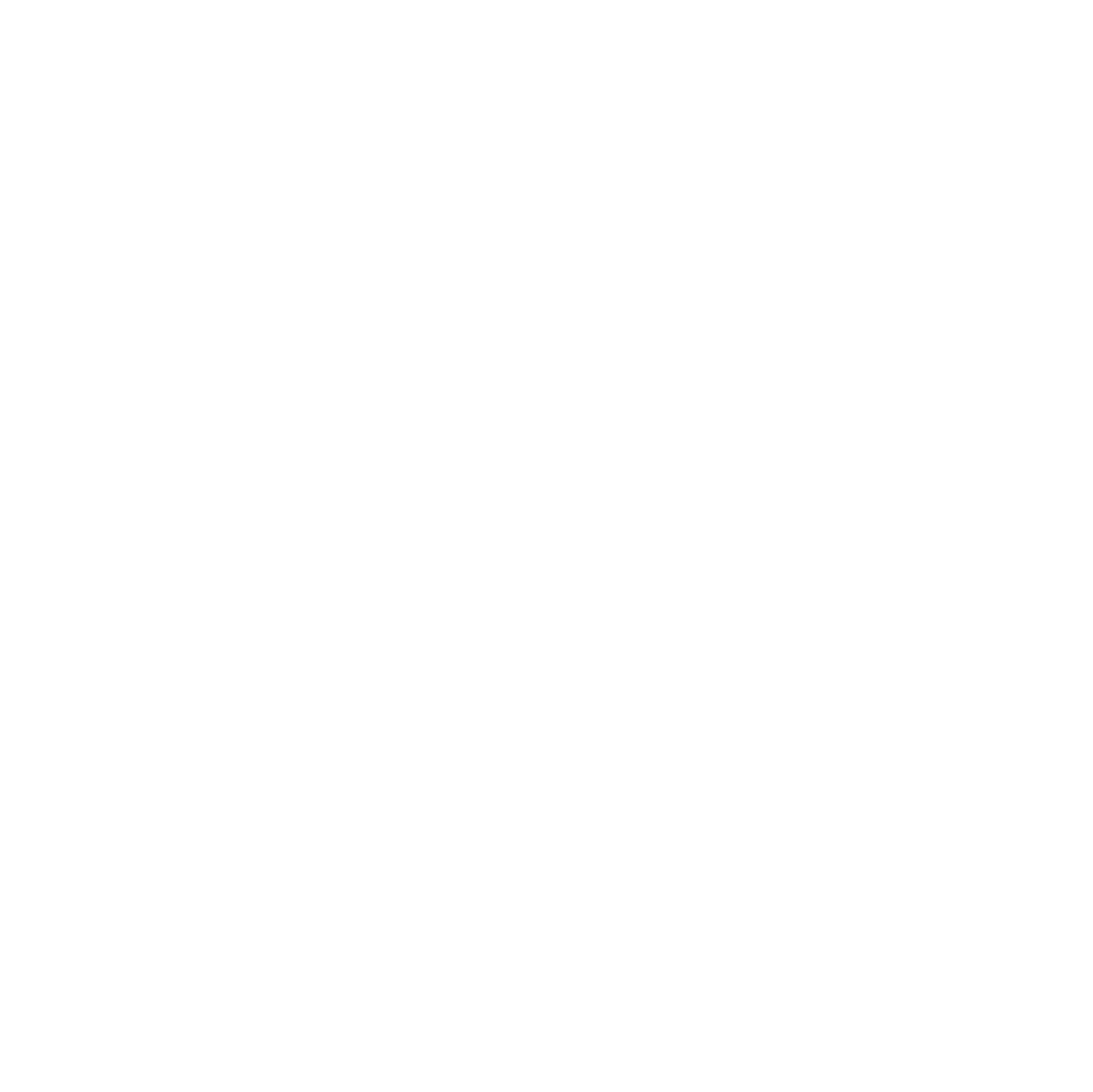
Engineered Nanotopographies Induce Transient Openings in the Nuclear Membrane
Sristilekha Nath

Also in the paleontology category:
Classification of dinosaur footprints using machine learning
Ryan Harrison

Hidden limbs in the “limbless skink” Brachymeles lukbani: developmental observations
Alexa Sadier
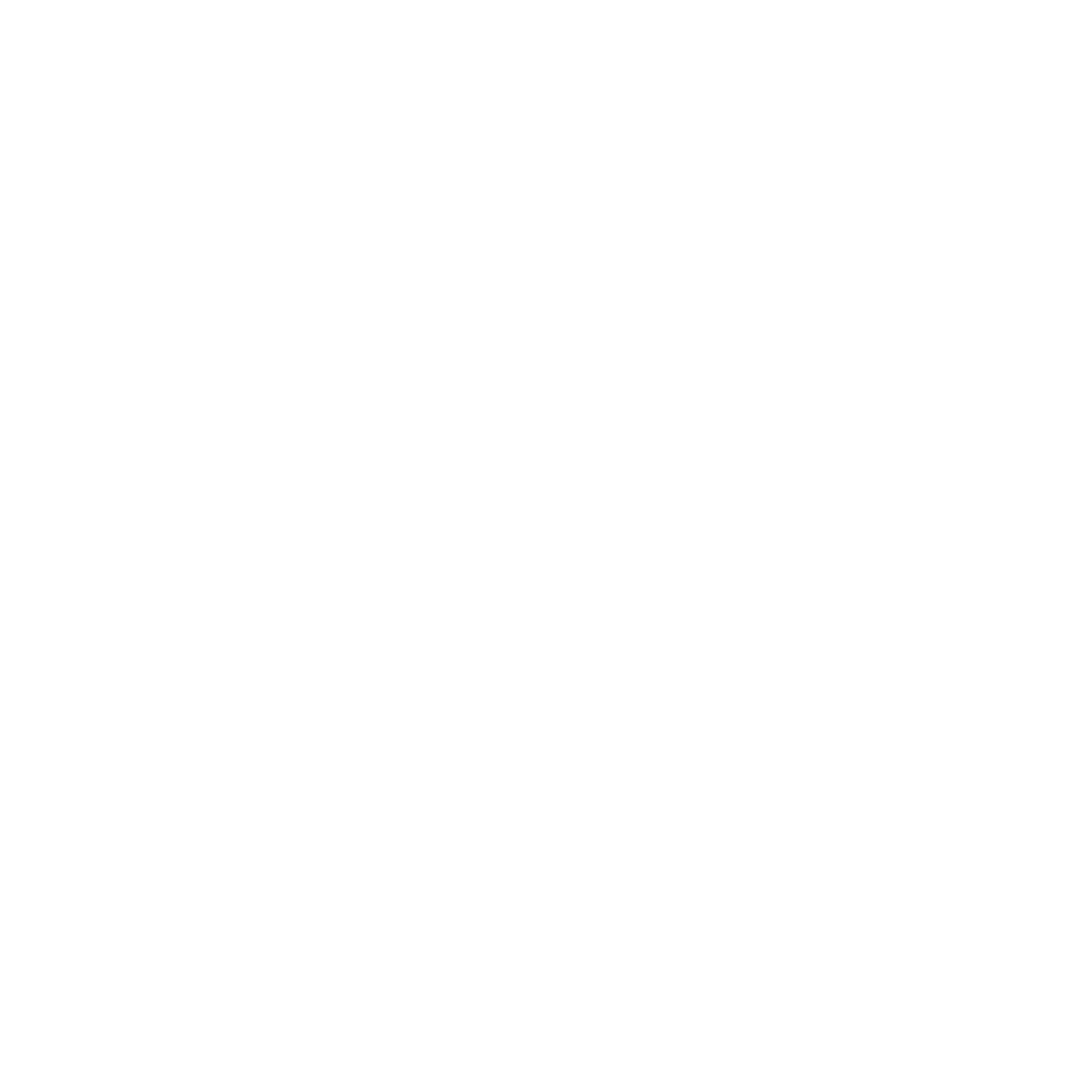
Soaring styles of extinct giant birds and pterosaurs
Sophia Friesen
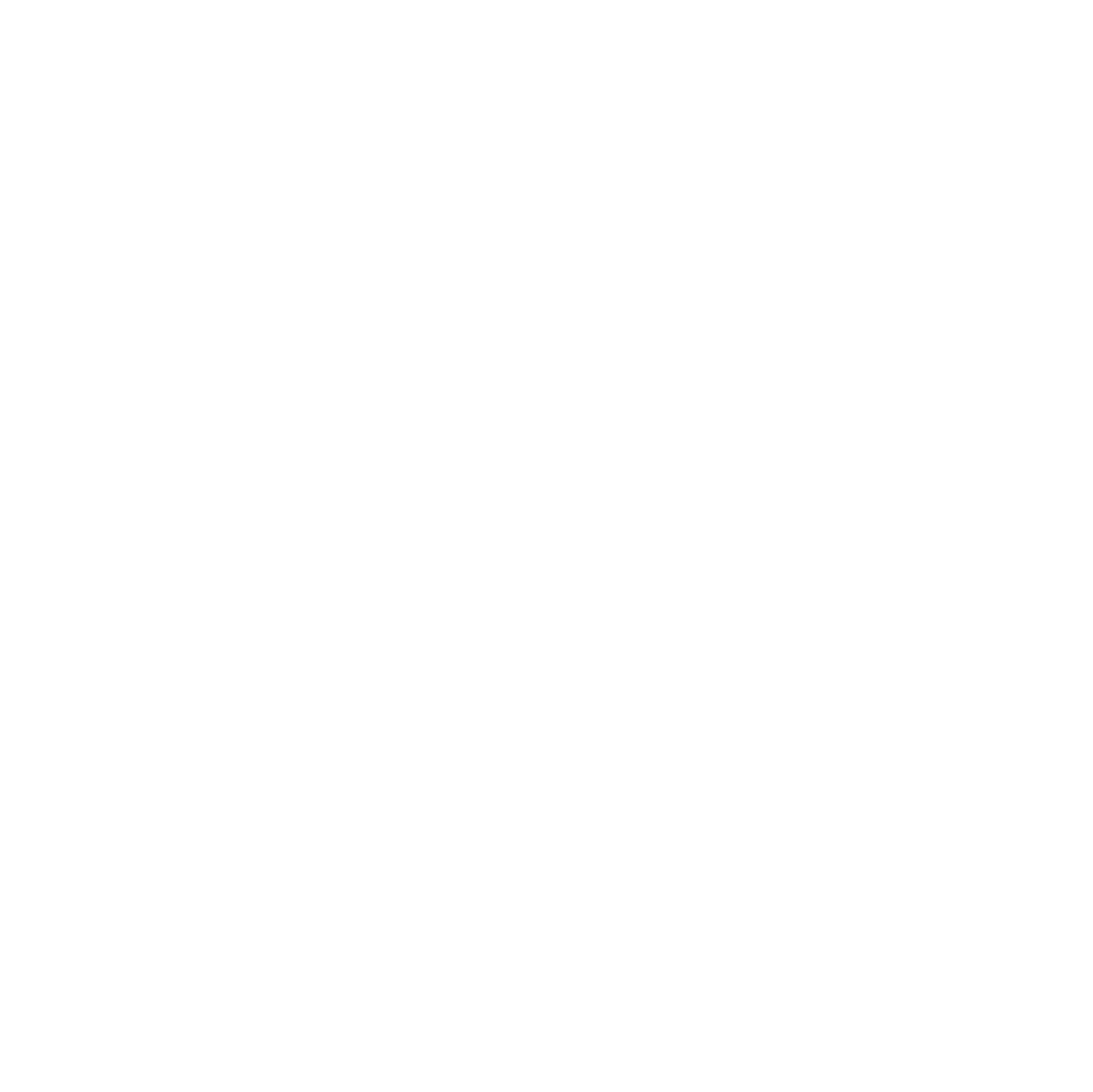
preListsanimal behavior and cognition category:
in the9th International Symposium on the Biology of Vertebrate Sex Determination
This preList contains preprints discussed during the 9th International Symposium on the Biology of Vertebrate Sex Determination. This conference was held in Kona, Hawaii from April 17th to 21st 2023.
List by | Martin Estermann |
Bats
A list of preprints dealing with the ecology, evolution and behavior of bats
List by | Baheerathan Murugavel |
FENS 2020
A collection of preprints presented during the virtual meeting of the Federation of European Neuroscience Societies (FENS) in 2020
List by | Ana Dorrego-Rivas |
Also in the biophysics category:
preLights peer support – preprints of interest
This is a preprint repository to organise the preprints and preLights covered through the 'preLights peer support' initiative.
List by | preLights peer support |
66th Biophysical Society Annual Meeting, 2022
Preprints presented at the 66th BPS Annual Meeting, Feb 19 - 23, 2022 (The below list is not exhaustive and the preprints are listed in no particular order.)
List by | Soni Mohapatra |
EMBL Synthetic Morphogenesis: From Gene Circuits to Tissue Architecture (2021)
A list of preprints mentioned at the #EESmorphoG virtual meeting in 2021.
List by | Alex Eve |
Biophysical Society Meeting 2020
Some preprints presented at the Biophysical Society Meeting 2020 in San Diego, USA.
List by | Tessa Sinnige |
ASCB EMBO Annual Meeting 2019
A collection of preprints presented at the 2019 ASCB EMBO Meeting in Washington, DC (December 7-11)
List by | Madhuja Samaddar et al. |
EMBL Seeing is Believing – Imaging the Molecular Processes of Life
Preprints discussed at the 2019 edition of Seeing is Believing, at EMBL Heidelberg from the 9th-12th October 2019
List by | Dey Lab |
Biomolecular NMR
Preprints related to the application and development of biomolecular NMR spectroscopy
List by | Reid Alderson |
Biophysical Society Annual Meeting 2019
Few of the preprints that were discussed in the recent BPS annual meeting at Baltimore, USA
List by | Joseph Jose Thottacherry |