The actin cortex acts as a mechanical memory of morphology in confined migrating cells
Posted on: 20 August 2024 , updated on: 23 August 2024
Preprint posted on 5 August 2024
“Cells "Remember" Their Shape via Actin Cortex, a Flexible Memory System”
Selected by Prasanna Padmanaban, Vibha SINGHCategories: biophysics
Background
Ever tried squeezing a soft couch through a narrow doorway? You have to squish and shape it to fit through, and once you’ve done so, the couch seems to “remember” that compressed shape for a bit, making it easier to fit through the door next time. Believe it or not, your cells do something similar when they move through tight spaces inside your body!
A fascinating new study by Yohalie Kalukula and her team (2024) shows that cells use a network of proteins called the actin cortex to help them remember their shape as they migrate through different environments. Just imagine the actin cortex as a flexible travel bag. When you pack this bag tightly for a trip, it reshapes itself to fit everything inside. The next time you need to pack for a similar trip, the bag “remembers” how to adjust its shape and makes the packing easier. The actin cortex works the same way for cells. It’s a flexible support network under the cell’s outer membrane that helps the cell remember its shape (acts as mechanical memory), especially when squeezing through tight spaces.
During both physiological and pathophysiological processes such as embryogenesis, wound healing and cancer metastasis, cells interact with heterogeneous surroundings and alter their shape and function, through lasting genetic changes. Recent studies highlight that cells maintain mechanical memory after prolonged exposure to constraints. However, it has not been investigated whether cells retain a mechanical memory after repetitive short-term confinement, which is relevant in the context of cellular navigation in various processes. This mechanical memory is especially important when cells are navigating narrow, confined spaces, like the tiny gaps between tissues. In this preprint, the authors describe how actin cortex remodelling acts as mechanical memory after multiple rounds of confinement exposure.
A person squishing a couch through a narrow doorway, analogous to a cell crossing a narrow bridge. The right image shows the time sequence of failed and successful crossings of cells (reproduced with authors permission).
Key Findings
(a) Morphological switches that involve symmetry breaking enable efficient navigation. This study describes that in a confined environment, compact cells migrate more efficiently than elongated cells. Compact morphology displayed greater retrograde actin flow, with significantly distinct front-rear asymmetry, while elongated cells were symmetric. A positive correlation was observed between these traits and the speed of migrations, indicating that compact cells are more persistent. Further, the study also provides a model that reinforces the observation that compact cells have persistent migration, while elongated cells frequently reorient themselves.
(b) Geometry-induced morphological switching. Switching between compact and elongated shapes occurs when cells migrate through confined space, and the switching is controlled by the geometry of the confinement, for instance, bridge length. Cells establish a memory of morphology during transitions, with a robust tendency to preserve the same morphology in successive switches, confirming long-term adaptation of past geometry.
(c) Compact cells have a thick actin cortex, which functions as mechanical memory. To understand the mechanism behind the long-term morphological memory of compact migrating cells, the authors investigated and later ruled out the influence of extracellular matrix remodeling as a potential factor. While elongated cells have symmetric microtubules, compact cells have significantly more microtubules in the rear suggesting symmetry breaking. Interestingly, compacted cells have a two times thicker cortex in the rear, which is essential in regulating cellular tension, and hence could act as mechanical memory.
(d) Actin cortex integrity is key for mechanical memory. Microtubule depolymerization using nocodazole affected cell migration, however did not impair mechanical memory of compact cells. Notably, Latrunculin-B (actin inhibitor) and Y27632 (ROCK inhibitor), which weaken the actin cortex, led to low shape index, diminished success in crossing confined spaces, and reduced migration speed, emphasizing the crucial role of the actin cortex and ROCK in sustaining compact morphology.
What we like about this preprint
While mechanical memory in materials, like shape memory polymers, is well understood, uncovering the mechanical memory of cells presents an intriguing new frontier to explore. The identification of the actin cortex as the fundamental basis of mechanical memory, which is responsible for cells retaining morphological shape during confined migration, is fascinating. These insights not only deepen our understanding of adaptations during cellular migration but also have potential implications in theoretical models of cellular behavior through complex microenvironments.
Questions to the authors
(1) When cells transition between different types of microenvironment, do cells recalibrate their mechanical memory?
(2) In this study, cells often migrate and are tested in straight geometries, but can their mechanical memory be retained when navigating curved geometries?
(3) It has been shown that the transition between compact and elongated forms is driven by bridge length. What would happen if you introduced a gradient in bridge length by tapering the width along its length?
doi: https://doi.org/10.1242/prelights.38168
Read preprintSign up to customise the site to your preferences and to receive alerts
Register hereAlso in the biophysics category:
Restoring mechanophenotype reverts malignant properties of ECM-enriched vocal fold cancer
Teodora Piskova

Motor Clustering Enhances Kinesin-driven Vesicle Transport
Sharvari Pitke
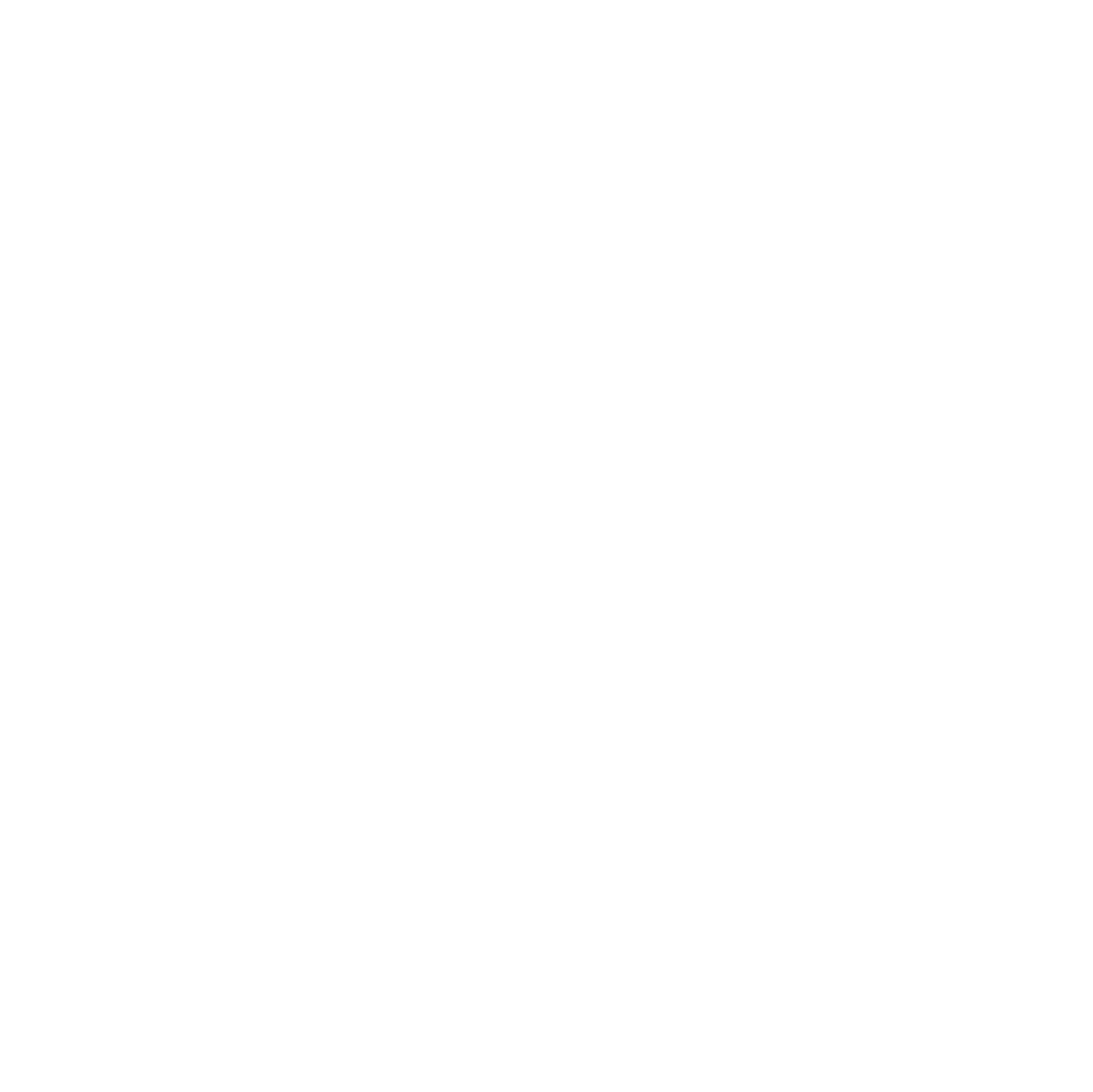
Global coordination of protrusive forces in migrating immune cells
yohalie kalukula
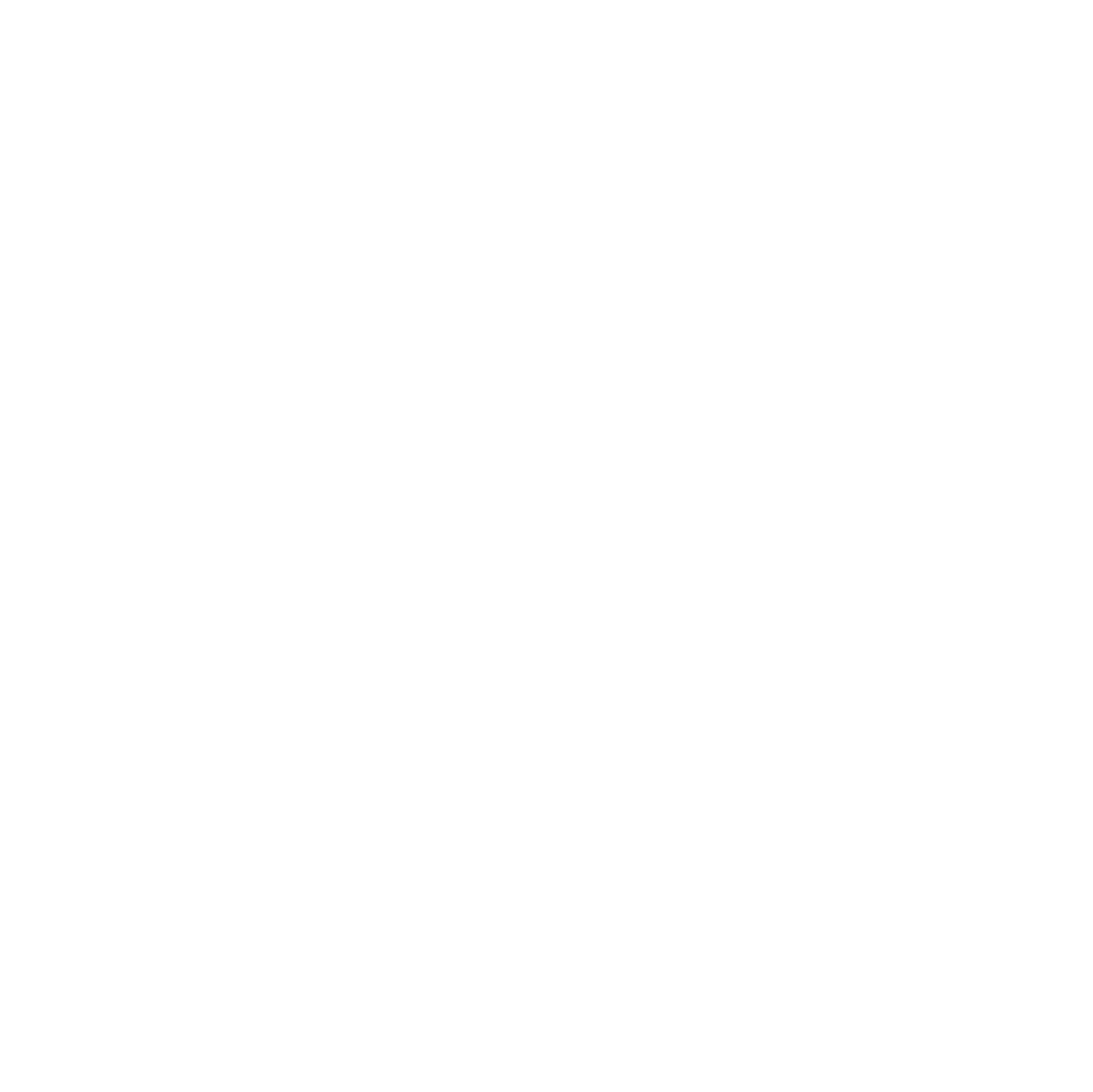
preListsbiophysics category:
in thepreLights peer support – preprints of interest
This is a preprint repository to organise the preprints and preLights covered through the 'preLights peer support' initiative.
List by | preLights peer support |
66th Biophysical Society Annual Meeting, 2022
Preprints presented at the 66th BPS Annual Meeting, Feb 19 - 23, 2022 (The below list is not exhaustive and the preprints are listed in no particular order.)
List by | Soni Mohapatra |
EMBL Synthetic Morphogenesis: From Gene Circuits to Tissue Architecture (2021)
A list of preprints mentioned at the #EESmorphoG virtual meeting in 2021.
List by | Alex Eve |
Biophysical Society Meeting 2020
Some preprints presented at the Biophysical Society Meeting 2020 in San Diego, USA.
List by | Tessa Sinnige |
ASCB EMBO Annual Meeting 2019
A collection of preprints presented at the 2019 ASCB EMBO Meeting in Washington, DC (December 7-11)
List by | Madhuja Samaddar et al. |
EMBL Seeing is Believing – Imaging the Molecular Processes of Life
Preprints discussed at the 2019 edition of Seeing is Believing, at EMBL Heidelberg from the 9th-12th October 2019
List by | Dey Lab |
Biomolecular NMR
Preprints related to the application and development of biomolecular NMR spectroscopy
List by | Reid Alderson |
Biophysical Society Annual Meeting 2019
Few of the preprints that were discussed in the recent BPS annual meeting at Baltimore, USA
List by | Joseph Jose Thottacherry |