Triclosan depletes the membrane potential in Pseudomonas aeruginosa biofilms inhibiting aminoglycoside induced adaptive resistance
Posted on: 27 April 2020 , updated on: 28 April 2020
Preprint posted on 14 April 2020
Article now published in PLOS Pathogens at http://dx.doi.org/10.1371/journal.ppat.1008529
Targeting antibiotic resistant bacteria in biofilms with synergistic killing methods
Selected by Amberley StephensCategories: microbiology, molecular biology
Background
The rise of antibiotic resistance and the formation of biofilms by Pseudomonas aeruginosa make them particularly hard to treat. The current treatment utilises the aminoglycoside family of antibiotics which cross the outer membrane, and inner membrane of this Gram-negative bacteria and inhibit protein translation by binding to the 30S subunit of the ribosome. P. aeruginosa form adaptive resistance to aminoglycosides by pumping them out of the cell using a type of efflux pump in the family called proton motive force (PMF) dependent resistance-nodule-cell division (RND) of efflux pumps. Despite the presence of these efflux pumps, aminoglycosides are still the first treatment for P. aeruginosa infections which are particularly prevalent in cystic fibrosis suffers.
Identifying new antimicrobials and getting them to market is a costly process both in time and money. Another approach is to find combination therapies which enhance the killing capacity of the antibiotics we currently have. This preprint explores the mechanism of a compound called triclosan which the authors show increases the killing effectivity of the aminoglycoside antibiotic tobramycin compared to tobramycin alone.
Results
Combination treatment is better than single compound treatment
Inner membrane permeabilization is one factor contributing to cell death by aminoglycosides. Initial treatment of P. aeruginosa in a biofilm form for 2 hours with an aminoglycoside called tobramycin lead to only 11% cell permeabilization. In combination with the compound triclosan cell permeabilization increased to 50%. Triclosan is a protonophore, an exciting group of compounds which act to move protons across lipid membranes and can disrupt membrane potentials (Figure 1). A protonophore, bedaquiline, was approved by the Food and Drug Administration in the USA as the newest drug in 40 years to target multi-drug resistant Mycobacterium tuberculosis[1,2]. Triclosan is also a known fatty acid synthesis inhibitor, yet Pseudomonas is inheritably resistant to triclosan and can even be selectively isolated from a mixed bacteria population with triclosan.
Figure 1. Protonophores are hydrophobic molecules containing hydrogen donor and acceptor groups which allow them to transport protons across lipid membranes. Figure created with BioRender.com.
Whole genome sequencing shows the aminoglycoside pulls the killing trigger
To investigate the synergistic mechanism and whether there was a dominant compound leading to cell death the authors purposefully induced resistance to identify mutations in key genes by gradually increasing the concentration of compounds over time. Whole genome sequencing identified single point mutations in a gene called fusA1 encoding EF-G1A, a protein involved in ribosome translocaion and recycling. This showed the main mode of cell killing was mediate by the aminoglycoside tobramycin.
Triclosan is only synergistic when aminoglycosides are present
Subsequently the authors investigated how triclosan was aiding the killing mechanism of tobramycin. Their previous work and high through put screening indicated that triclosan only enhanced killing when an aminoglycoside was present and the resistance mechanism, the efflux pump, was activated[3].
They tagged tobramycin with a fluorescent dye and incubated the P. aeruginosa biofilms with and without triclosan for 30 minutes, allowing time for the compounds to be internalised, but not to kill the cells. The bacteria were subsequently recovered and the quantity of tagged tobramycin released from the cells was quantified. The amount of tagged tobramycin released was higher from cells treated with triclosan than without, as the triclosan had prevented the efflux of the tobramycin leading to accumulation within the cells.
Triclosan disrupts the membrane potential that drives the RND efflux pump
RND efflux pumps utilise the proton gradient and move protons across the inner membrane in an opposite direction to the exported drug. The balance of protons across membranes creates a membrane potential (Δѱ). Protonophores can alter this membrane potential due to their ability to accept and donate protons and transfer them across lipid membranes (Figure 2).
To investigate changes in Δѱ in the presence of triclosan the P. aeruginosa bacteria were treated with a dye sensitive to Δѱ, DiOC2(3) dye. When untreated, 20% of cells maintained their Δѱ, when treated with triclosan this reduced to only 5%. When treated with tobramycin this increased to 37% due to other mechanisms increasing Δѱ and leading to increased activity of the efflux pumps. Yet treatment with both compounds lead to ~10% of cells with maintained Δѱ.
A derivative, methyl-triclosan without a dissociable proton did not have the same effects as triclosan, cells maintained their Δѱ and efflux pump activity, therefore showing the photonophore moiety of triclosan is key to its function.
Figure 2. Schematic of the synergistic mechanism of action of tobramycin and triclosan and the mechanism of adaptive resistance. 1. Tobramycin crosses cell membranes and inhibits translation by binding to ribosomes inside the bacteria. 2. The RND-type efflux pump is responsible for adaptive resistance and is formed of three main components, the outer membrane proteins (OMP), the membrane fusion proteins (MFP) and the inner membrane proteins (IMP) which work together to pump aminoglycosides out of the cell while pumping protons (H+) into the cytosol. 3. Triclosan disrupts the proton gradient by transferring protons into the cytosol. This prevents the functioning of the efflux pump and allows tobramycin concentrations to increase in the cytosol leading to cell death. Taken from Figure 7 in preprint.
A combination of triclosan and tobramycin is effective in reducing P. aeruginosa in wounds in vivo
To test the effect of synergistic combination treatment in vivo, mice with open wounds were infected with bioluminescent P. aeruginosa. A hydrogel of triclosan only, tobramycin only and a combination of triclosan and tobramycin was applied. The combination treatment lead to a 4-fold decrease in P. aeruginosa when imaged (Figure 3.). Tobramycin alone lead to a 2.5 fold decrease and triclosan alone lead to no decrease in infection.
Figure 3. Treatment with a triclosan and tobramycin loaded hydrogel lead to a decrease in P. aeruginosa infection. The luminescent P. aeruginosa in a wound model were imaged before and after treatment with a hydrogel containing triclosan and tobramycin. The black arrow indicates where the hydrogel was placed on the wound. A decrease in luminescence radiance indicated a reduction in live bacteria after treatment.
Conclusion
The addition of the photonophore, triclosan, with the aminoglycoside tobramycin to treat P. aeruginosa biofilm infection lead to a 99% increase in cell death in vitro after 6-hrs of treatment and a reduction of P. aeruginosa infection in an in vivo model compared to tobramycin alone. The mechanism of action was determined to based upon the protonphore property of triclosan which transports protons across the inner membrane into the cytosol. This disrupts the proton gradient that the RND-efflux pump relies on to pump out the tobramycin. The result is a greater accumulation of the tobramycin inside the cell, arrest of translation as the drug binds to ribosomes, and eventually cell death.
Why I chose this preprint
The ability of bacteria to resist antibiotics is both fascinating and scary. I’d never heard of a protonophores before so I wanted to learn more about their mechanism of action. The paper had a good flow during reading and well thought out experiments that followed a logical order.
Questions for authors
Have you tested these compounds on bacteria not in biofilm formation? Does it make a difference?
How is this different/better than oxyclozanide which is also mentioned in the preprint as a protonophore uncoupler?
How far into biofilm do these compounds penetrate? Does it make a difference how mature the biofilm is as to how effective the compounds are?
Why are hydrogels used to administer the treatment? How do you determine how much compound is taken into the hydrogel?
Will hydrogels likely be used to treat wound infected humans?
References
- Andries K, Verhasselt P, Guillemont J, Göhlmann HWH, Neefs JM, Winkler H, Van Gestel J, Timmerman P, Zhu M, Lee E, et al.: A diarylquinoline drug active on the ATP synthase of Mycobacterium tuberculosis. Science (80- ) 2005, 307:223–227.
- Mahajan R: Bedaquiline: First FDA-approved tuberculosis drug in 40 years. Int J Appl Basic Med Res 2013, 3:1.
- Maiden MM, Agostinho Hunt AM, Zachos MP, Gibson JA, Hurwitz ME, Mulks MH, Waters CM: Triclosan is an aminoglycoside adjuvant for eradication of pseudomonas aeruginosa biofilms. Antimicrob Agents Chemother 2018, 62.
doi: https://doi.org/10.1242/prelights.19407
Read preprintSign up to customise the site to your preferences and to receive alerts
Register hereAlso in the microbiology category:
Characterization of natural product inhibitors of quorum sensing in Pseudomonas aeruginosa reveals competitive inhibition of RhlR by ortho-vanillin
UofA IMB565 et al.

Feedback loop regulation between viperin and viral hemorrhagic septicemia virus through competing protein degradation pathways
UofA IMB565 et al.
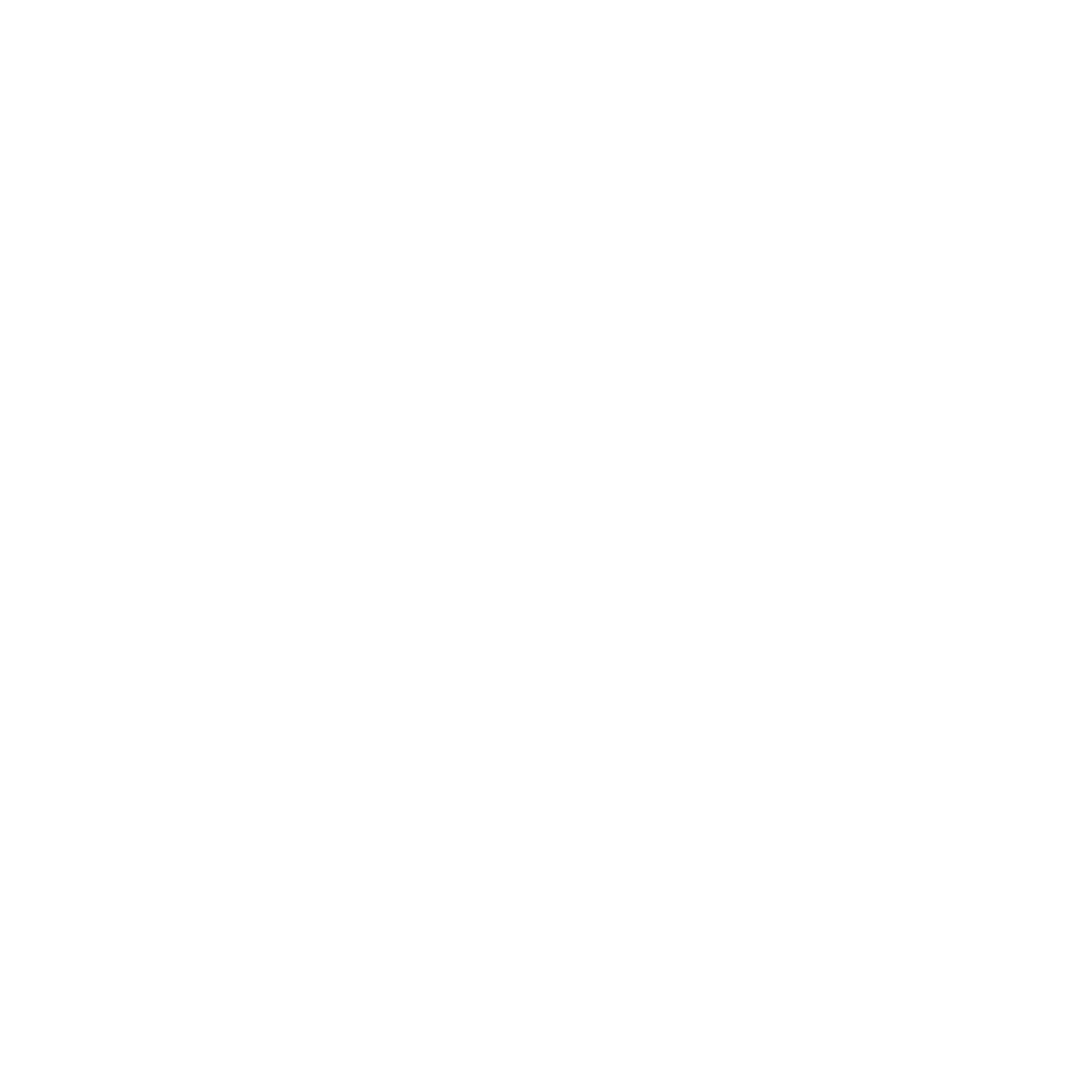
Lytic bacteriophages interact with respiratory epithelial cells and induce the secretion of antiviral and proinflammatory cytokines
UofA IMB565 et al.

Also in the molecular biology category:
Cell cycle-dependent mRNA localization in P-bodies
Mohammed JALLOH

Notch3 is a genetic modifier of NODAL signalling for patterning asymmetry during mouse heart looping
Bhaval Parmar
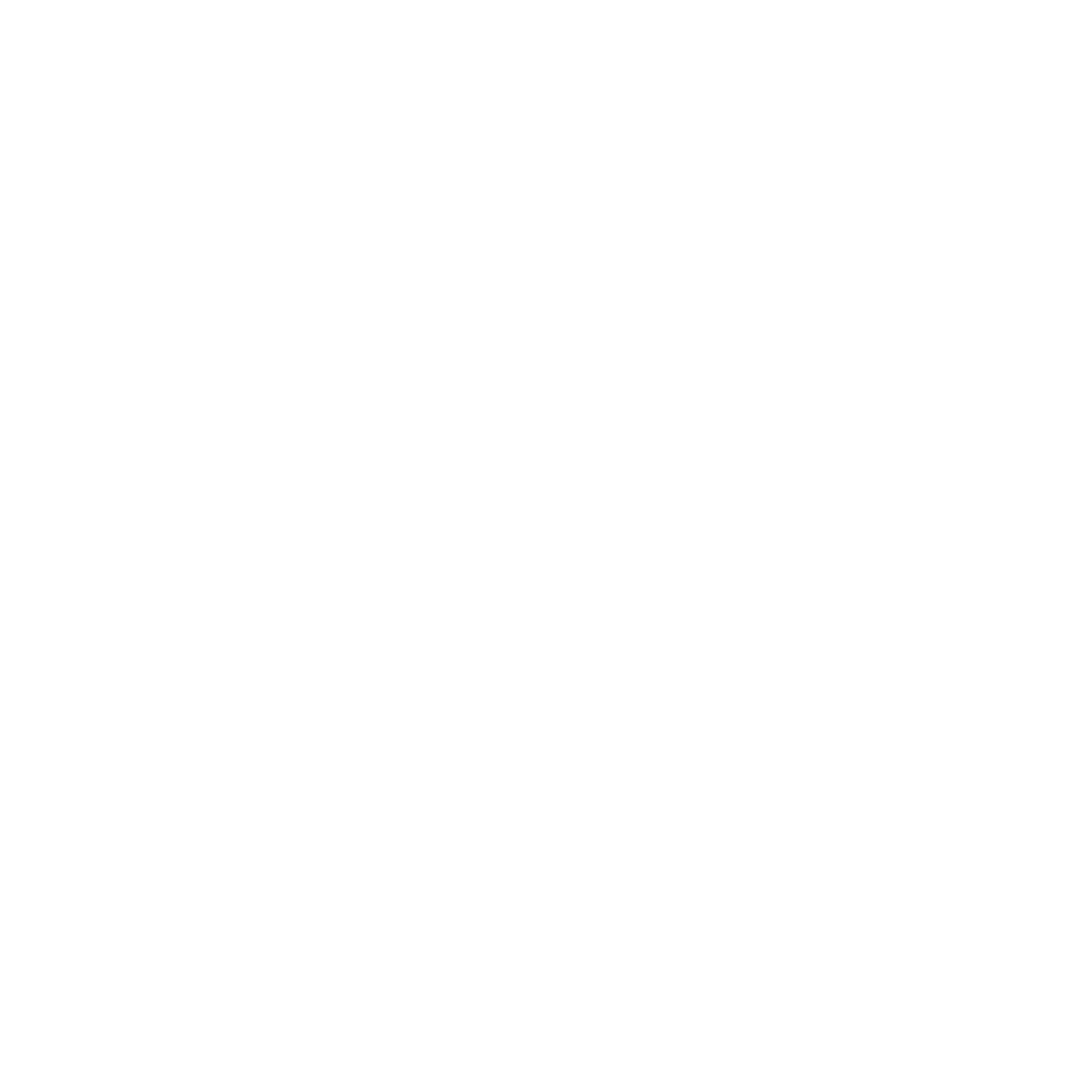
Fetal brain response to maternal inflammation requires microglia
Manuel Lessi

preListsmicrobiology category:
in theBioMalPar XVI: Biology and Pathology of the Malaria Parasite
[under construction] Preprints presented at the (fully virtual) EMBL BioMalPar XVI, 17-18 May 2020 #emblmalaria
List by | Dey Lab, Samantha Seah |
1
ECFG15 – Fungal biology
Preprints presented at 15th European Conference on Fungal Genetics 17-20 February 2020 Rome
List by | Hiral Shah |
EMBL Seeing is Believing – Imaging the Molecular Processes of Life
Preprints discussed at the 2019 edition of Seeing is Believing, at EMBL Heidelberg from the 9th-12th October 2019
List by | Dey Lab |
Antimicrobials: Discovery, clinical use, and development of resistance
Preprints that describe the discovery of new antimicrobials and any improvements made regarding their clinical use. Includes preprints that detail the factors affecting antimicrobial selection and the development of antimicrobial resistance.
List by | Zhang-He Goh |
Also in the molecular biology category:
BSCB-Biochemical Society 2024 Cell Migration meeting
This preList features preprints that were discussed and presented during the BSCB-Biochemical Society 2024 Cell Migration meeting in Birmingham, UK in April 2024. Kindly put together by Sara Morais da Silva, Reviews Editor at Journal of Cell Science.
List by | Reinier Prosee |
‘In preprints’ from Development 2022-2023
A list of the preprints featured in Development's 'In preprints' articles between 2022-2023
List by | Alex Eve, Katherine Brown |
CSHL 87th Symposium: Stem Cells
Preprints mentioned by speakers at the #CSHLsymp23
List by | Alex Eve |
9th International Symposium on the Biology of Vertebrate Sex Determination
This preList contains preprints discussed during the 9th International Symposium on the Biology of Vertebrate Sex Determination. This conference was held in Kona, Hawaii from April 17th to 21st 2023.
List by | Martin Estermann |
Alumni picks – preLights 5th Birthday
This preList contains preprints that were picked and highlighted by preLights Alumni - an initiative that was set up to mark preLights 5th birthday. More entries will follow throughout February and March 2023.
List by | Sergio Menchero et al. |
CellBio 2022 – An ASCB/EMBO Meeting
This preLists features preprints that were discussed and presented during the CellBio 2022 meeting in Washington, DC in December 2022.
List by | Nadja Hümpfer et al. |
EMBL Synthetic Morphogenesis: From Gene Circuits to Tissue Architecture (2021)
A list of preprints mentioned at the #EESmorphoG virtual meeting in 2021.
List by | Alex Eve |
FENS 2020
A collection of preprints presented during the virtual meeting of the Federation of European Neuroscience Societies (FENS) in 2020
List by | Ana Dorrego-Rivas |
ECFG15 – Fungal biology
Preprints presented at 15th European Conference on Fungal Genetics 17-20 February 2020 Rome
List by | Hiral Shah |
ASCB EMBO Annual Meeting 2019
A collection of preprints presented at the 2019 ASCB EMBO Meeting in Washington, DC (December 7-11)
List by | Madhuja Samaddar et al. |
Lung Disease and Regeneration
This preprint list compiles highlights from the field of lung biology.
List by | Rob Hynds |
MitoList
This list of preprints is focused on work expanding our knowledge on mitochondria in any organism, tissue or cell type, from the normal biology to the pathology.
List by | Sandra Franco Iborra |