I am a computational biologist who enjoys exploring cell signalling through data-driven mathematical modelling. I am especially interested in understanding the dynamic interplay of systems components and how information is specifically processed and linked to cell-fate decisions despite cross-talk and noise. Recently graduated from the joint Master’s programme in Molecular Techniques in Life Science in Stockholm, Sweden, I am a PhD candidate in Evangelia Petsalaki’s research group (Whole Cell Signalling) at the European Bioinformatics Institute and the University of Cambridge as part of the EMBL International PhD Programme.
My PhD project involves the development of context-specific executable models of signalling processes. For this the current plan is to optimise an existing method in my group that derives phenotype/context-specific networks from omics data and develop a method that can translate these into executable models. Besides science, I am addicted to all sorts of ballsports (tennis, badminton, table tennis, foosball) and enjoy multi-day hiking and kayaking tours .
Finding the right words to evaluate research: An empirical appraisal of eLife’s assessment vocabulary
Benjamin Dominik Maier
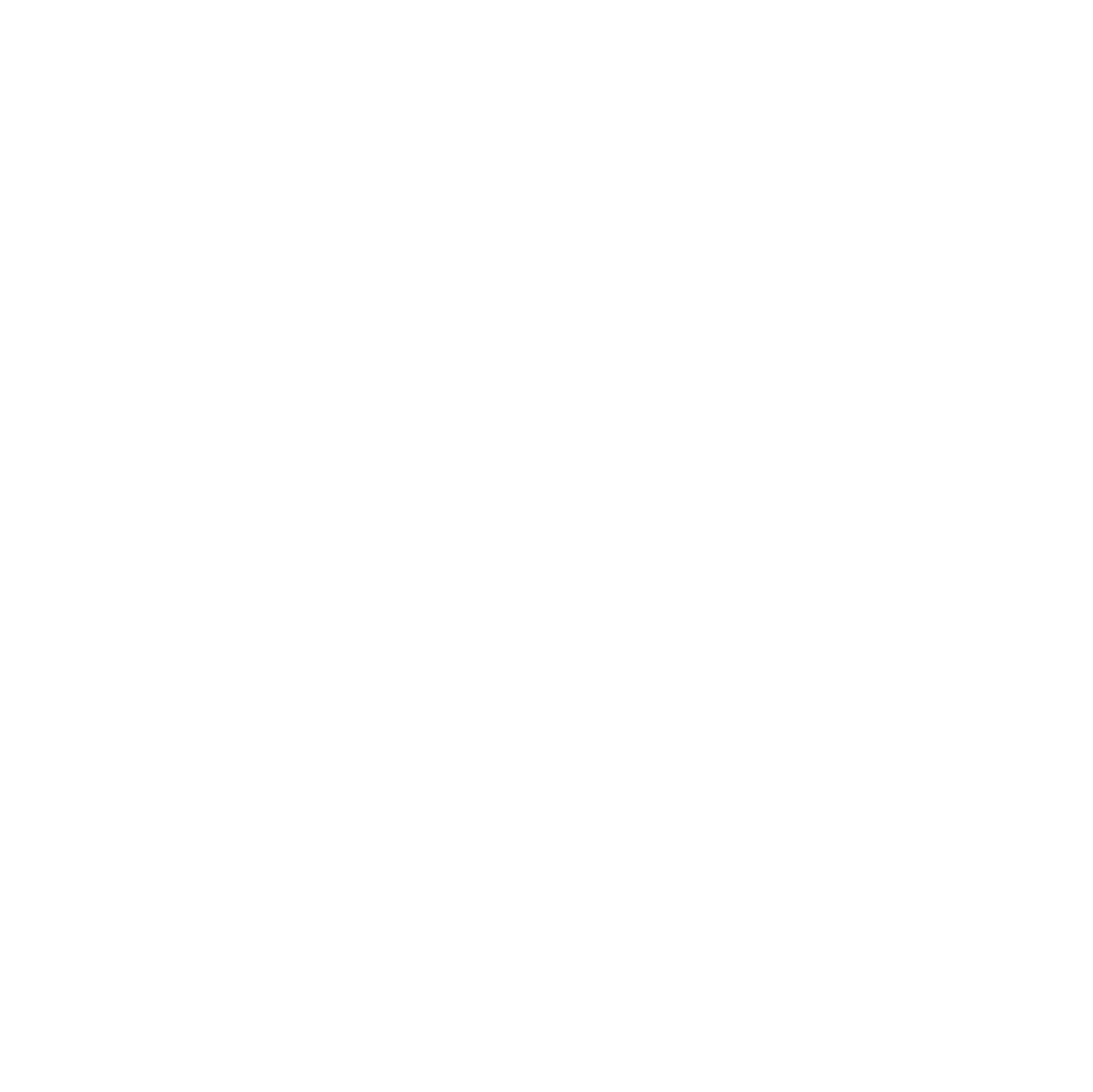
Expressive modeling and fast simulation for dynamic compartments
Benjamin Dominik Maier
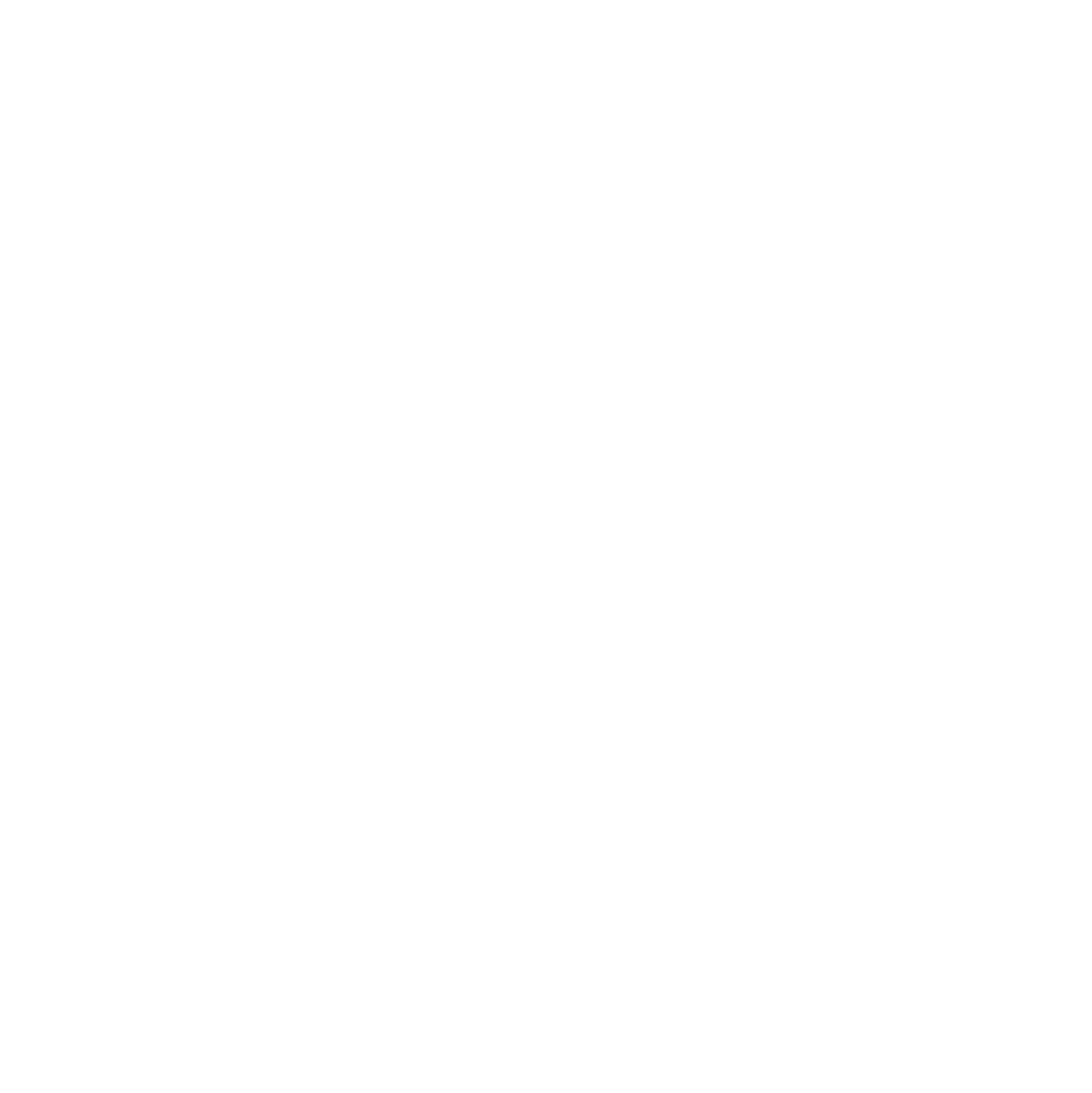
Holimap: an accurate and efficient method for solving stochastic gene network dynamics
Benjamin Dominik Maier
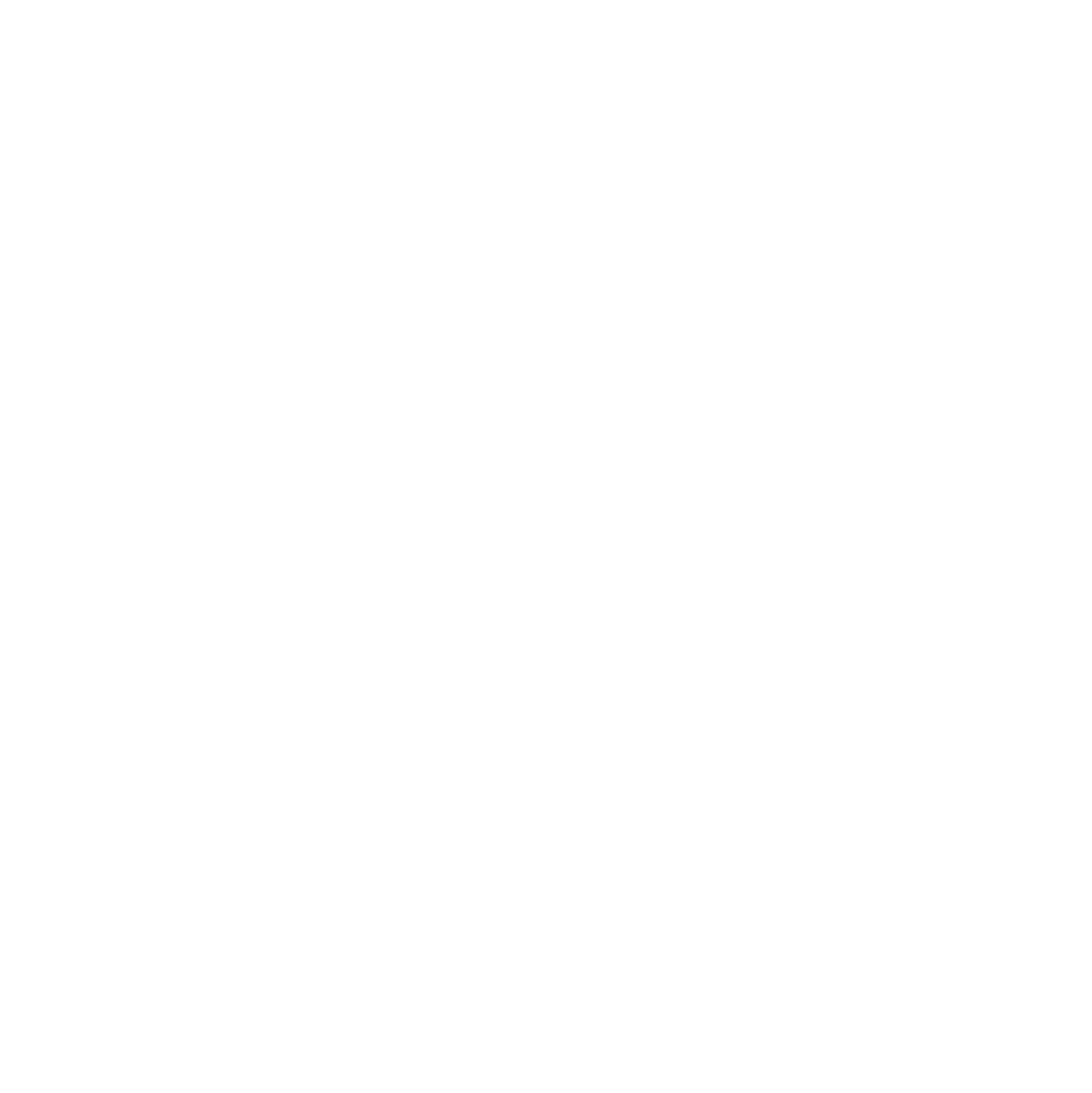
Digital Microbe: A Genome-Informed Data Integration Framework for Collaborative Research on Emerging Model Organisms
Benjamin Dominik Maier, Jennifer Ann Black

Inferring protein from mRNA concentrations using convolutional neural networks
Benjamin Dominik Maier

Patterned embryonic invagination evolved in response to mechanical instability
AND
Divergent evolutionary strategies preempt tissue collision in fly gastrulation
Reinier Prosee et al.
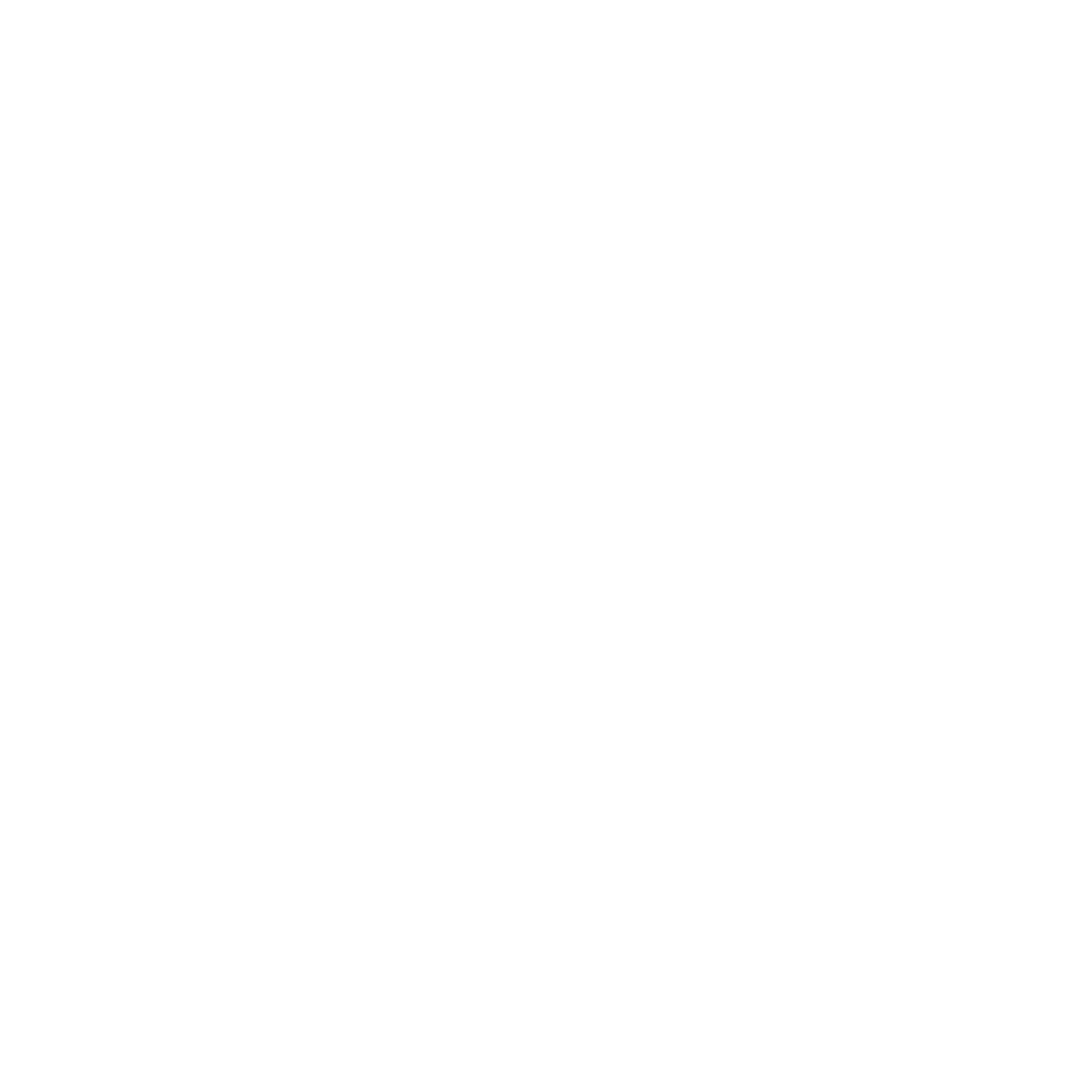
Multi-pass, single-molecule nanopore reading of long protein strands with single-amino acid sensitivity
Benjamin Dominik Maier, Samantha Seah

Inference of drug off-target effects on cellular signaling using Interactome-Based Deep Learning
Benjamin Dominik Maier

Digitize your Biology! Modeling multicellular systems through interpretable cell behavior
Benjamin Dominik Maier et al.
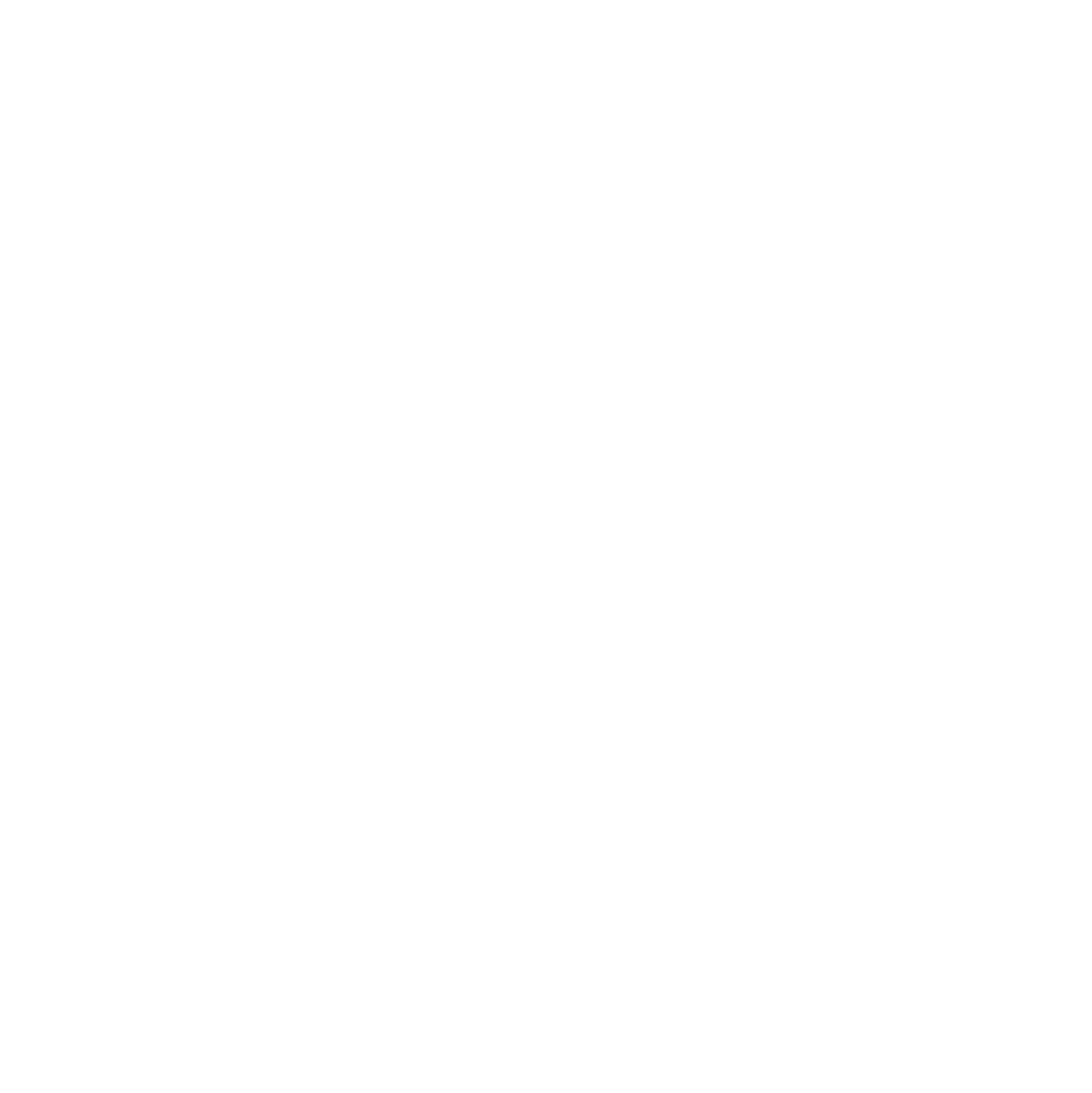
A Phosphoproteomics Data Resource for Systems-level Modeling of Kinase Signaling Networks
Benjamin Dominik Maier
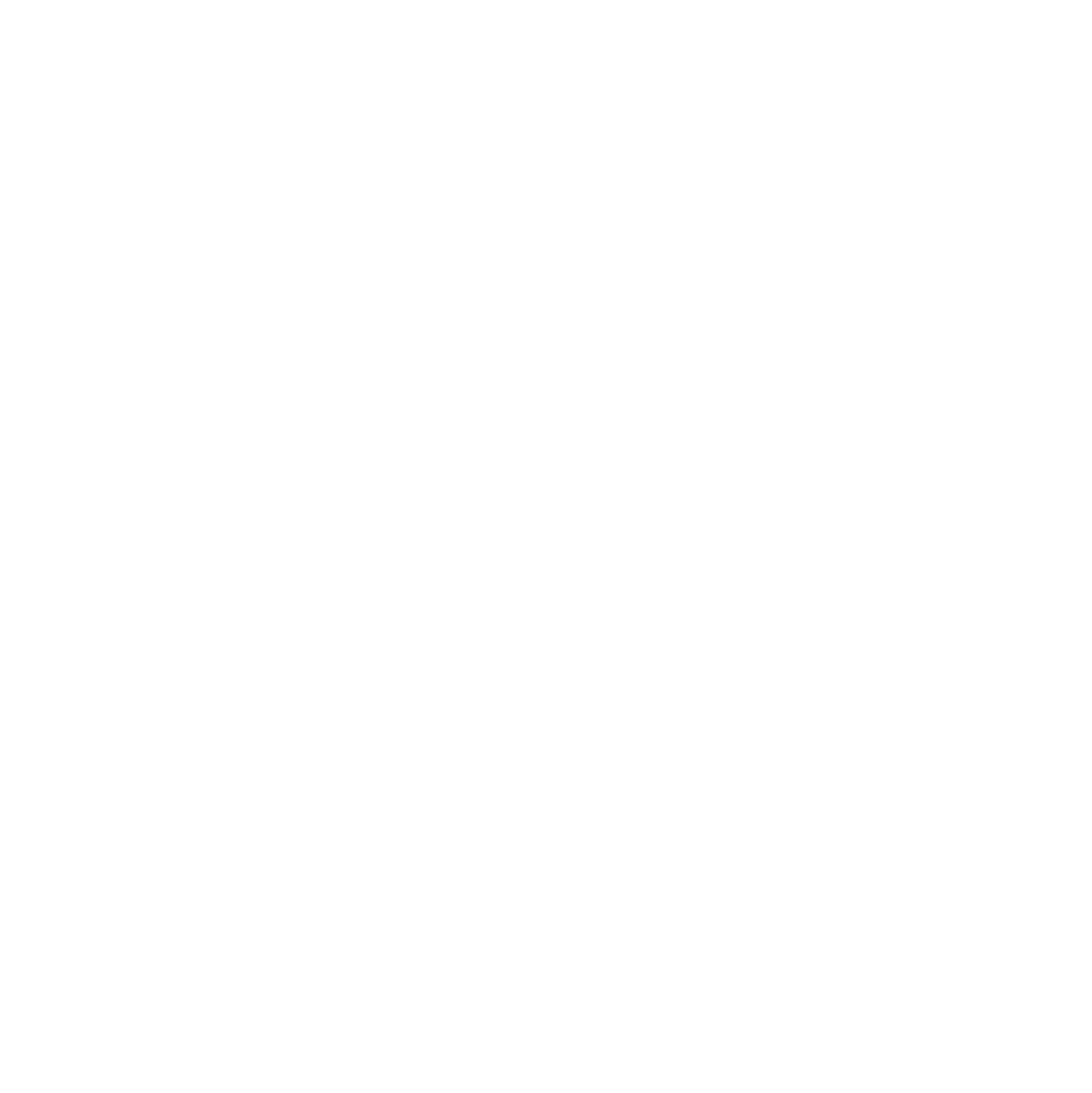
Similarity metric learning on perturbational datasets improves functional identification of perturbations
Benjamin Dominik Maier, Anna Foix Romero

Biologically informed NeuralODEs for genome-wide regulatory dynamics
Benjamin Dominik Maier

Inference of drug off-target effects on cellular signaling using Interactome-Based Deep Learning
Congratulations to Nikolaos Meimetis, Douglas A. Lauffenburger and Avlant Nilsson! Their preprint has now been published in iScience (link to the article). Although the manuscript remained largely the same, notable changes include an expanded lethality analysis and the addition of a new section exploring prediction performance across drug classes further improving the already worth-reading article.…
Posted on | 29 April 2024 |
Biologically informed NeuralODEs for genome-wide regulatory dynamics
Congratulations to Intekhab Hossain, Viola Fanfani, Jonas Fischer, John Quackenbush and Rebekka Burkholz! Their manuscript has been published in Genome Biology on May 21st 2024 (link to the journal article). Jonas Fischer has been added to the author list during the review process and contributed to this work with the newly added B cell modeling…
Posted on | 22 May 2024 |
A data-driven Boolean model explains memory subsets and evolution in CD8+ T cell exhaustion
Congratulations to Geena V. Ildefonso and Stacey D. Finley! Their preprint has been published in npj systems biology and applications on July 31st 2023 (link to the article: https://www.nature.com/articles/s41540-023-00297-2). Overall, there are no substantial differences between the peer-reviewed journal article and the preprint article. Addressing one of my questions raised in the preLight (Q1), the…
Posted on | 17 August 2023 |
Intekhab Hossain (ihossain@g.harvard.edu), the first author of this study, is happy to answer questions and guide users through the setup process of PHOENIX, so feel free to send him a message or comment here 🙂
Supplement: Current Approaches to Study Protein-Protein Interactions
*Genetic*
– Yeast Two-Hybrid (Y2H) System involves fusing one protein of interest to a DNA-binding domain (BDB) and another protein to an activation domain (AD) (Fields and Song, 1989). If the two proteins interact, the DNA-binding and activation domains come into close proximity, leading to the expression of a reporter gene. Newer versions of the assay (Weile et al., 2017; Luck et al. 2020) allow for screening of entire proteomes or random peptide libraries, which can enable unbiased identification of novel interactions.
– Protein fragment complementation assay (PCA) involves splitting a protein of interest into two fragments and fusing each fragment with a complementary protein fragment (Michnick et al., 2007). The resulting fusion proteins can only reconstitute the original protein activity if the two proteins interact and bring the two fragments together. This approach can be used to study protein-protein interactions in vivo and has been used for high-throughput screening to identify potential drug targets.
– Protein-array based methods involve immobilising large numbers of purified proteins on a solid surface (e.g. glass slide). The arrays can then be probed with (fluorescently) labelled proteins of interest to identify potential interaction partners. This approach enables the simultaneous screening of thousands of protein pairs, but it does necessitate laborious protein purification steps.
*Biophysical*
– Fluorescence/Bioluminescence Resonance Energy Transfer (FRET/BRET) involves labelling one protein with a donor fluorophore/luminescence and the other protein with an acceptor fluorophore (Sun et al., 2016). When the two proteins interact, the donor fluorophore transfers energy to the acceptor fluorophore, resulting in fluorescence, which can be measured by a detector.
– Mass spectrometry-based methods involve the fractionation of cell lysates by size or other physicochemical properties, followed by mass spectrometry analysis to identify co-eluting proteins (Richards et al., 2021). Although this approach can identify numerous potential interaction partners in an unbiased manner, it can be quite laborious since it requires protein purification steps.
*Biochemical*
– Immunoprecipitation-based methods such as Co-Immunoprecipitation (Co-IP) or Tandem Affinity Purification (TAP) involve the isolation of protein-protein interactions by adsorbing the protein complex onto beads using a combination of protein tags and specific antibodies (Lin & Lai, 2017), and subsequently identifying them by mass spectrometry or Western blotting.
– Surface Plasmon Resonance (SPR) involves immobilising one protein on a chip and flowing the other protein over it. The binding between the two proteins is measured in real-time based on the changes in the refractive index of the chip.
– Proximity-dependent labelling methods such as BioID and APEX enable identification of interacting proteins within a certain distance of the protein of interest and can be used to identify both stable and transient interactions in an unbiased way (Chen & Perrimon, 2017).
*Computational*
– Co-evolution Analysis makes inferences about protein-protein interactions using alignments (both sequence and structure) and phylogenetic distances. The approach can be used to predict functional residues and domains involved in the PPI.
– Molecular Docking Analysis uses structural templates of individual proteins to predict the structure of a complex. The approach is commonly used to screen large libraries of small molecule compounds for potential drug candidates that can target PPIs.
While most models require experimentally determined interactions as prior knowledge, there are also some approaches to predict interactions de novo.
Supplemental References
Chen, C. L., & Perrimon, N. (2017). Proximity‐dependent labeling methods for proteomic profiling in living cells. WIREs Developmental Biology, 6(4). https://doi.org/10.1002/wdev.272
Fields, S., & Song, O. (1989). A novel genetic system to detect protein-protein interactions. Nature, 340(6230), 245–246. https://doi.org/10.1038/340245a0
Lin, J. S., & Lai, E. M. (2017). Protein-Protein Interactions: Co-Immunoprecipitation. Methods in molecular biology (Clifton, N.J.), 1615, 211–219. https://doi.org/10.1007/978-1-4939-7033-9_17
Luck, K., Kim, DK., Lambourne, L. et al. A reference map of the human binary protein interactome. Nature 580, 402–408 (2020). https://doi.org/10.1038/s41586-020-2188-x
Michnick, S., Ear, P., Manderson, E. et al. (2007) Universal strategies in research and drug discovery based on protein-fragment complementation assays. Nat Rev Drug Discov, 6, 569–582. https://doi.org/10.1038/nrd2311
Richards, A.L., Eckhardt, M. and Krogan, N.J. (2021) Mass spectrometry‐based protein–protein interaction networks for the study of human diseases, Molecular Systems Biology, 17(1). https://doi.org/10.15252/msb.20188792
Sun, S., Yang, X., Wang, Y., & Shen, X. (2016). In Vivo Analysis of Protein–Protein Interactions with Bioluminescence Resonance Energy Transfer (BRET): Progress and Prospects. International Journal of Molecular Sciences, 17(10), 1704. https://doi.org/10.3390/ijms17101704
Weile, J., Sun, S., Cote, A. G., Knapp, J., Verby, M., Mellor, J. C., … Roth, F. P. (2017). A framework for exhaustively mapping functional missense variants. Molecular Systems Biology, 13(12), 957. https://www.doi.org/10.15252/msb.20177908
3 months
Benjamin Dominik Maier
The preprint has now also been highlighted in a Nature Technology Feature about the latest advances in Nanopore sequencing technologies: https://www.nature.com/articles/d41586-024-01280-5