Cancer modeling by Transgene Electroporation in Adult Zebrafish (TEAZ)
Posted on: 3 May 2018 , updated on: 22 June 2018
Preprint posted on 9 April 2018
Article now published in Disease Models & Mechanisms at http://dx.doi.org/10.1242/dmm.034561
TEAZing fish melanoma models – Transgene Electroporation of Adult Zebrafish as a simple way of genetically modifying somatic tissues
Selected by Hannah BrunsdonCategories: cancer biology, genetics
Background
Zebrafish are proving themselves very useful as a model of complex pathologies, including melanoma. Researchers have generated numerous zebrafish transgenic melanoma model, which harbour mutations commonly found in human melanoma patients. These models are powerful tools that allow investigation into the molecular mechanisms behind melanoma, and crucially, into the discovery of drugs to target and kill cancerous cells.
These transgenic models are not perfect however, as often the timing and location of tumour formation can be quite variable[1], making monitoring of carcinogenesis amongst cohorts tricky. Tumour transplantation studies give more spatial and temporal control, but there is often a requirement to use immunosuppressed animals which may differ from immunocompetent fish in terms of the tumour microenvironment[2].
To address these problems, Callahan and colleagues have established a technique called TEAZ (Transgene Electroporation in Adult Zebrafish) in which different oncogenic transgenic constructs are electroporated directly into adult somatic tissue either simultaneously or sequentially to model melanoma.
TEAZ in a nutshell: plasmid DNA, in this case a ubb:tdTomato construct that will ubiquitously drive fluorescent protein expression, is injected into the adult zebrafish. Brief electrical pulses are applied to the anaesthetised fish to allow the plasmid to enter cells. The fluorescent protein is then observable using fluorescent microscopy. From Callahan et al., 2018 Figure 1a (under CC-BY-NC-ND 4.0)
Key findings
- The authors first electroporated multiple constructs to drive fluorescent protein expression in defined regions of the zebrafish. Not only was fluorescence still stable after eight months, but cell type-specific transgene expression was achieved by using a melanocyte-specific reporter construct (mitfa:tdTomato).
- The team then took an existing melanoma model (mitfa:BRAFV600E;p53-/-;mitfa-/-)[3], and electroporated in CRISPR-Cas9 elements to disrupt the tumour suppressor rb1. Strikingly, the electroporated animals developed melanotic lesions in just 3-7 weeks, compared to the 3-6 months taken for non-electroporated fish.
- These melanotic lesions were highly tumourigenic, and reminiscent of human high-grade melanoma. Histological analyses confirmed distant metastases in a range of locations – an event not documented with previous melanoma models.
- As proof-of-principle, the team electroporated mitfa:tdTomato into an existing tumour, in order to see if established tumours could be sequentially modified. In one week, cell-specific fluorescence could be observed within the tumour, suggesting that TEAZ could be used to model secondary mutations after tumour onset.
Why I chose this preprint
As someone in a zebrafish disease model lab, I often get a ‘lightbulb moment’ where I think of a great experiment, followed by a sinking feeling as I realise that it would only be made possible by using my fish’s great-grandchildren after rounds of painstaking genotyping. A way of introducing transgenes into an adult fish in any genetic background, and having spatial and temporal control of this could save labs so much time and reduce the numbers of fish required.
As our understanding of the molecular events behind cancer grows, it is important that our disease models reflect the nuances of human pathologies. Which genes drive tumour initiation vs tumour metastasis, and does the order of mutation acquisition matter? The thought of being able to reproducibly disrupt or repair genes in adult somatic cells using CRISPR, and to thereby screen for factors influencing tumour progression and metastasis is really exciting, and I look forward to seeing what this team and others do with TEAZ next. I also think TEAZ could be broadly useful as a quick way to introduce multiple fluorescent reporter constructs into a disease model, in order to image different subpopulations of cells in vivo within their microenvironment.
Open questions
- The analysis of a tumour from an rb1 sgRNA-electroporated fish revealed the formation of a mixed histology sarcoma and melanoma tumour. The authors attributed this to using a ubiquitous instead of tissue-specific Cas9. As only two electroporated fish were used for this study, it might be important to find out how often these unexpected tumours occur in a larger cohort relative to more typical melanomas. If using a tissue-specific Cas9, the sgRNA and Cas9 might modify a much smaller proportion of cells, so it would be interesting to see if melanomas form to the same extent as when using ubiquitously-expressed Cas9.
- Perhaps related to the above, Callahan and colleagues sequenced these tumour cells and found two different mutations in 2% of rb1 alleles each. This was evidently more than enough to drive aggressive melanomas, but it will be interesting to see whether this frequency might be too low to make a biological difference when investigating other genes, or whether this mosaicism is in fact a good thing as it is more representative of a malignant subpopulation of cells within a tumour.
References
- Patton, E. E., et al. (2005). BRAF mutations are sufficient to promote nevi formation and cooperate with p53 in the genesis of melanoma. Curr. Biol. 15 , 249–254.
- Tang, Q., et al. (2016). Imaging tumour cell heterogeneity following cell transplantation into optically clear immune-deficient zebrafish. Nat. Commun. 7 , 10358.
- Ceol, C. J., et al. (2011). The histone methyltransferase SETDB1 is recurrently amplified in melanoma and accelerates its onset. Nature 471 , 513–517.
Sign up to customise the site to your preferences and to receive alerts
Register hereAlso in the cancer biology category:
Mitochondria-derived nuclear ATP surge protects against confinement-induced proliferation defects
Teodora Piskova

Spatial transcriptomics elucidates medulla niche supporting germinal center response in myasthenia gravis thymoma
Jessica Chevallier
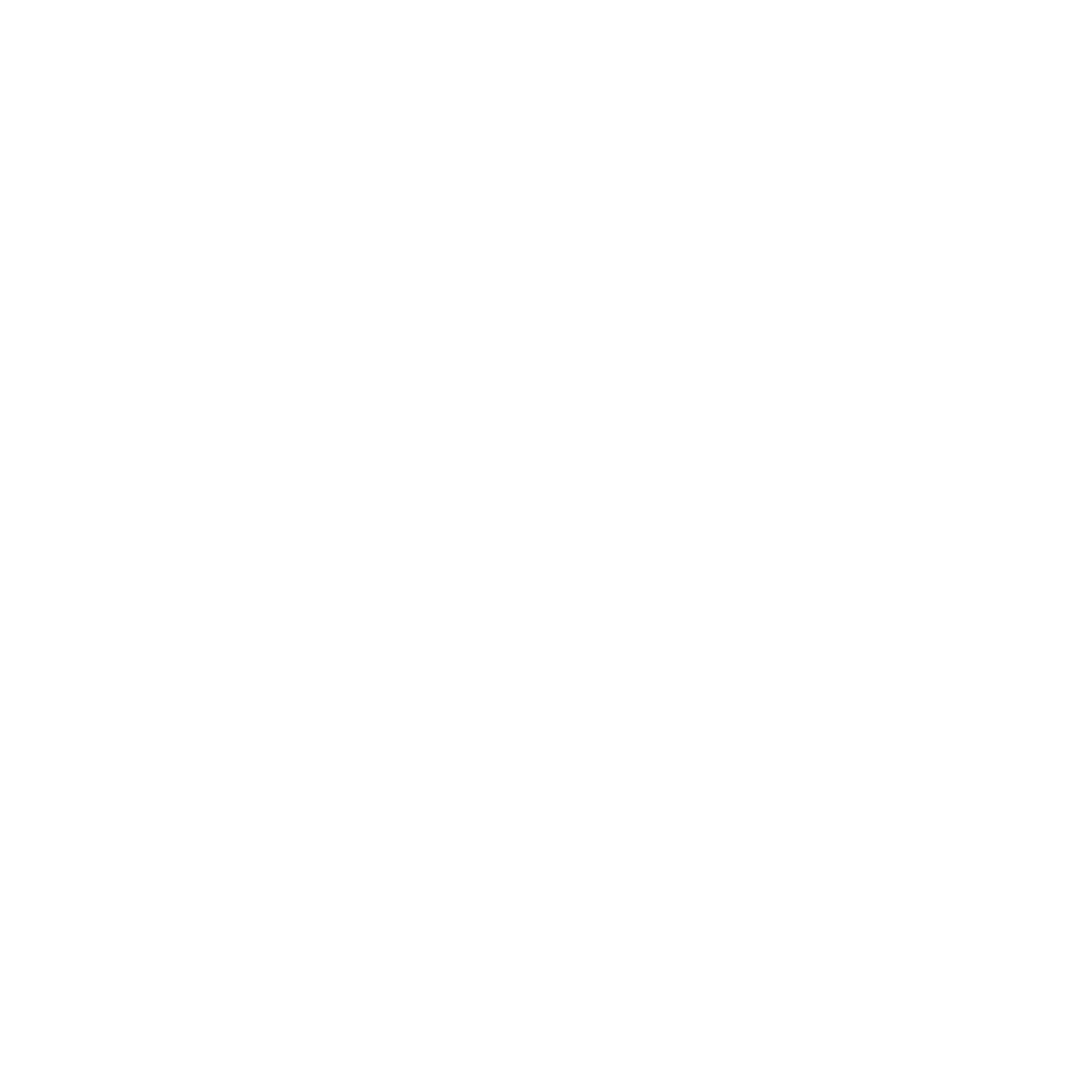
Invasion of glioma cells through confined space requires membrane tension regulation and mechano-electrical coupling via Plexin-B2
Jade Chan

Also in the genetics category:
Enhancer-driven cell type comparison reveals similarities between the mammalian and bird pallium
Rodrigo Senovilla-Ganzo

Lipid-Based Transfection of Zebrafish Embryos: A Robust Protocol for Nucleic Acid Delivery
Roberto Rodríguez-Morales
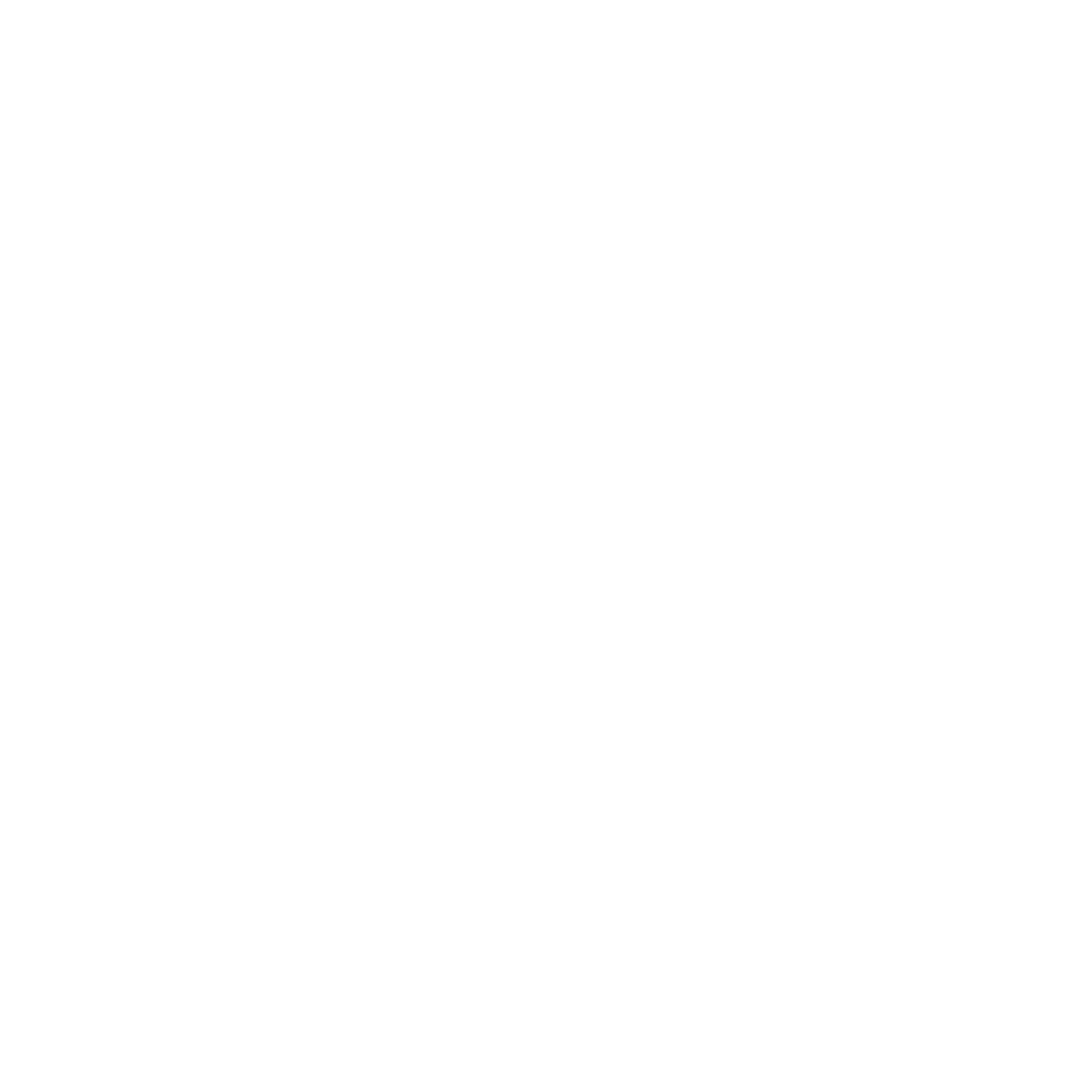
Temporal constraints on enhancer usage shape the regulation of limb gene transcription
María Mariner-Faulí
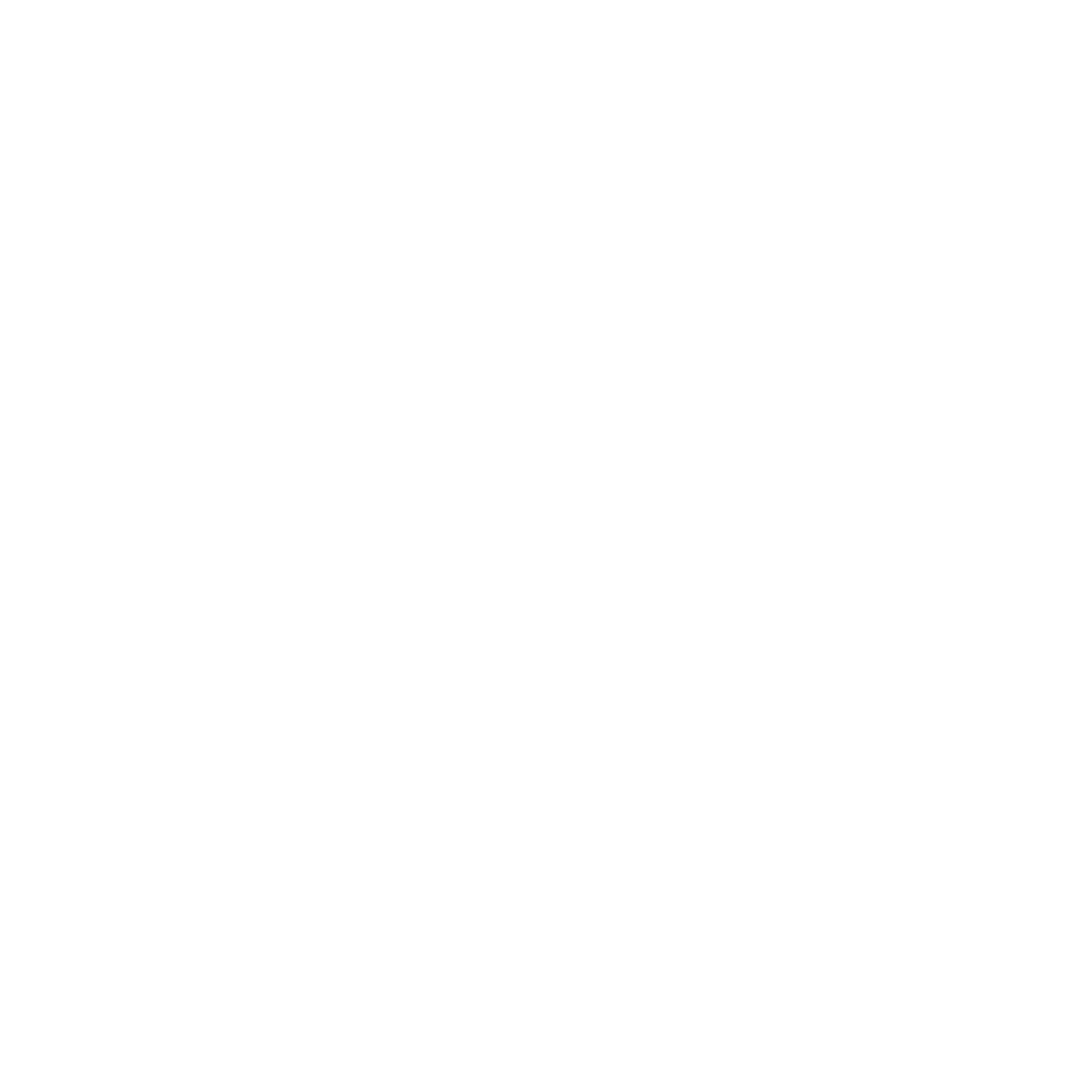
preListscancer biology category:
in theBSCB-Biochemical Society 2024 Cell Migration meeting
This preList features preprints that were discussed and presented during the BSCB-Biochemical Society 2024 Cell Migration meeting in Birmingham, UK in April 2024. Kindly put together by Sara Morais da Silva, Reviews Editor at Journal of Cell Science.
List by | Reinier Prosee |
CSHL 87th Symposium: Stem Cells
Preprints mentioned by speakers at the #CSHLsymp23
List by | Alex Eve |
Journal of Cell Science meeting ‘Imaging Cell Dynamics’
This preList highlights the preprints discussed at the JCS meeting 'Imaging Cell Dynamics'. The meeting was held from 14 - 17 May 2023 in Lisbon, Portugal and was organised by Erika Holzbaur, Jennifer Lippincott-Schwartz, Rob Parton and Michael Way.
List by | Helen Zenner |
CellBio 2022 – An ASCB/EMBO Meeting
This preLists features preprints that were discussed and presented during the CellBio 2022 meeting in Washington, DC in December 2022.
List by | Nadja Hümpfer et al. |
Fibroblasts
The advances in fibroblast biology preList explores the recent discoveries and preprints of the fibroblast world. Get ready to immerse yourself with this list created for fibroblasts aficionados and lovers, and beyond. Here, my goal is to include preprints of fibroblast biology, heterogeneity, fate, extracellular matrix, behavior, topography, single-cell atlases, spatial transcriptomics, and their matrix!
List by | Osvaldo Contreras |
Single Cell Biology 2020
A list of preprints mentioned at the Wellcome Genome Campus Single Cell Biology 2020 meeting.
List by | Alex Eve |
ASCB EMBO Annual Meeting 2019
A collection of preprints presented at the 2019 ASCB EMBO Meeting in Washington, DC (December 7-11)
List by | Madhuja Samaddar et al. |
Lung Disease and Regeneration
This preprint list compiles highlights from the field of lung biology.
List by | Rob Hynds |
Anticancer agents: Discovery and clinical use
Preprints that describe the discovery of anticancer agents and their clinical use. Includes both small molecules and macromolecules like biologics.
List by | Zhang-He Goh |
Biophysical Society Annual Meeting 2019
Few of the preprints that were discussed in the recent BPS annual meeting at Baltimore, USA
List by | Joseph Jose Thottacherry |
Also in the genetics category:
BSDB/GenSoc Spring Meeting 2024
A list of preprints highlighted at the British Society for Developmental Biology and Genetics Society joint Spring meeting 2024 at Warwick, UK.
List by | Joyce Yu, Katherine Brown |
BSCB-Biochemical Society 2024 Cell Migration meeting
This preList features preprints that were discussed and presented during the BSCB-Biochemical Society 2024 Cell Migration meeting in Birmingham, UK in April 2024. Kindly put together by Sara Morais da Silva, Reviews Editor at Journal of Cell Science.
List by | Reinier Prosee |
9th International Symposium on the Biology of Vertebrate Sex Determination
This preList contains preprints discussed during the 9th International Symposium on the Biology of Vertebrate Sex Determination. This conference was held in Kona, Hawaii from April 17th to 21st 2023.
List by | Martin Estermann |
Alumni picks – preLights 5th Birthday
This preList contains preprints that were picked and highlighted by preLights Alumni - an initiative that was set up to mark preLights 5th birthday. More entries will follow throughout February and March 2023.
List by | Sergio Menchero et al. |
Semmelweis Symposium 2022: 40th anniversary of international medical education at Semmelweis University
This preList contains preprints discussed during the 'Semmelweis Symposium 2022' (7-9 November), organised around the 40th anniversary of international medical education at Semmelweis University covering a wide range of topics.
List by | Nándor Lipták |
20th “Genetics Workshops in Hungary”, Szeged (25th, September)
In this annual conference, Hungarian geneticists, biochemists and biotechnologists presented their works. Link: http://group.szbk.u-szeged.hu/minikonf/archive/prg2021.pdf
List by | Nándor Lipták |
2nd Conference of the Visegrád Group Society for Developmental Biology
Preprints from the 2nd Conference of the Visegrád Group Society for Developmental Biology (2-5 September, 2021, Szeged, Hungary)
List by | Nándor Lipták |
EMBL Conference: From functional genomics to systems biology
Preprints presented at the virtual EMBL conference "from functional genomics and systems biology", 16-19 November 2020
List by | Jesus Victorino |
TAGC 2020
Preprints recently presented at the virtual Allied Genetics Conference, April 22-26, 2020. #TAGC20
List by | Maiko Kitaoka et al. |
ECFG15 – Fungal biology
Preprints presented at 15th European Conference on Fungal Genetics 17-20 February 2020 Rome
List by | Hiral Shah |
Autophagy
Preprints on autophagy and lysosomal degradation and its role in neurodegeneration and disease. Includes molecular mechanisms, upstream signalling and regulation as well as studies on pharmaceutical interventions to upregulate the process.
List by | Sandra Malmgren Hill |
Zebrafish immunology
A compilation of cutting-edge research that uses the zebrafish as a model system to elucidate novel immunological mechanisms in health and disease.
List by | Shikha Nayar |