Directed Evolution of Enzymes based on in vitro Programmable Self-Replication
Posted on: 4 June 2021 , updated on: 7 June 2021
Preprint posted on 22 April 2021
High-throughput directed evolution of a DNA nicking enzyme through programmable DNA networks identified improved variants with higher thermal stability or catalytic rate
Selected by Soni MohapatraCategories: bioengineering, synthetic biology
Background
Directed evolution is a Nobel prize winning strategy that allowed scientists to engineer enzymes in a test tube (1). The strategy is being employed widely to design enzymes that can either function in non-physiological conditions, show altered substrate specificities, or display novel/enhanced activities. These tailor-made enzymes have found a place in therapeutics, research, biotechnology, and other industries. The first step of this approach involves generating a protein library where different alterations are introduced into the protein sequence-of-interest. These alterations can be introduced through random/focused mutagenesis, protein domain insertion, or chimeric protein production to name a few (2). However, fishing out protein sequences from the generated library with desirable functions can often be like a “needle in a haystack” situation. The second step of the directed evolution approach therefore requires designing an appropriate screening strategy that enriches for optimized protein variants. In contrast to in vivo selection assays, in vitro selection assays do not suffer from low transformation efficiency or mutations in host chromosomes that could affect selection unexpectedly. In vitro selection assays also allow evolving enzymes under selective pressures and for functionalities, that may not be typically feasible in vivo.
One such popular in vitro selection approach, CSR (Compartmentalized Self Replication) takes advantage of the linkage between genes and gene products without using cells (3). CSR is particularly useful for directed evolution of DNA polymerases as only functional DNA polymerase variants that can efficiently replicate their encoding gene in an emulsion PCR are enriched. Recent development of CPR (Compartmentalized Partnered Replication) by Ellefson et al. (4) has expanded the range of compartmentalized in vitro selection approaches beyond DNA polymerases. In this preprint, the authors constructed molecular circuitry for enrichment of nicking enzyme libraries of specific variants under different selective pressures pushing the boundaries of CSR further.
Key findings
Programmable DNA network based Compartmentalized Self-Replication:
Dramé-Maigné et al. developed a DNA based molecular network for linking the activity of a DNA nicking enzyme to the replication of its encoding gene through a programmed feedback loop. Nt.BstNBI (NBI) was chosen as the target because of its wide-spread usage in biotechnological industries in its natural form. Bacteria expressing a library of mutagenized NBI variants are encapsulated in emulsion droplets. The molecular program consists of three modules. The first module senses the activity of the NBI variant and generates short oligos linearly over time. The second module amplifies the incoming signal from the first module and exponentially generates the amount of DNA strands necessary to trigger the third module. The third and the final primer-generating module generates forward and reverse primers for amplification of the encoding gene, and the amount of primers generated via this molecular program is correlated with the activity of the nicking enzyme. Genes encoding for the active nickase variants get enriched in the selection pool by virtue of the availability of sufficient primers for PCR amplification of encoding gene sequence. The authors name this in vitro, screening-free approach Programmable External Network-based Compartmentalized Self-Replication (PEN CSR).
This high-throughput method screens up to ten million gene variants simultaneously, with the reported selection efficiency of the selection procedure very close to the theoretical maximum.
Deep mutational scanning for unraveling the fitness landscape of proteins
Current usage of NBI in molecular diagnostics and other biotechnological applications are in its natural form. Having NBI variants with increased catalytic rate and higher thermal stability are desirable for improving the applicability of NBI. The authors exposed the protein to two different kinds of selective pressure: kinetic stress, by reducing incubation time for primer amplification, and thermal stress, by applying a heat shock at 65 C. They were able to identify favorable mutations necessary for optimal functioning of the protein under the applied selective pressure. The majority of the mutations that performed better under kinetic stress were primarily clustered around the DNA binding pocket of the nickase. While these mutations can be rationalized based on the available crystal structures, other identified favorable mutations were distributed over surface exposed residues. This suggests that structural studies of proteins are not always sufficient to identify residues imminent to the functioning of the protein and a deep mutational scanning of proteins is necessary for a thorough understanding of the fitness landscape of proteins.
Why I like the preprint
In this article, the authors demonstrated how molecular circuitries can be designed for directed evolution of enzymes under different selective pressures. They set up a molecular circuitry consisting of three modules, that translates the activity of NBI into primer concentration. I particularly liked the usage of a non-linear module that fastens the generation of input signal for triggering the primer-generating module.
Their method extended the usage of compartmentalized self-replication beyond DNA polymerases. The implementation of molecular networks for directed evolution also allows for selective pressure conditions and functionalities that are typically not possible in living cells. I look forward to seeing how this method will be adapted for other different kinds of enzymes.
Future directions
Compartmentalized in vitro selection is a very efficient directed evolution strategy, but it requires developing the right selection assay that enriches for enzymes with the desired functionalities. However, such selection strategies have so far been mostly limited to DNA polymerases. In this work, the authors constructed a DNA-based synthetic network to set up a feedback loop linking the enzymatic activity of a DNA nickase to the replication of its encoding gene. Powered by next-generation sequencing, this method can aid in exploration of the mutational and fitness landscape of enzymes under different selective pressures. In the future, other programmable networks can be similarly adapted for other DNA interacting enzymes that will expand the scope of this platform.
Questions/comments for the authors
- It would be interesting to see how PEN CSR can be used to evolve a wide diversity of enzymes. In combination with cell-free protein expression systems and/or reverse transcription systems, PEN CSR has the potential to be useful beyond DNA processing I would love to know what the authors think the future of PEN CSR entails.
- I wonder why shorter incubation times for primer amplification led to a greater selectivity of active gene fraction over the inactive
- Is it possible that mutations resulting in higher non-specific nickase activity lead to higher self-selection? Do the authors plan to improve the current molecular program to enrich for nickases with higher specificity?
References
- Frances H. Arnold (2018). Directed evolution: Bringing new chemistry to life. Angew Chem. 57,
- Packer et al. (2015). Methods for the Directed Evolution of Proteins. Nature Reviews Genetics 16,
- Ghadessy et al. (2001). Directed evolution of polymerase function by compartmentalized self-replication. PNAS 98,
- Ellefson et al. (2014). Directed evolution of genetic parts and circuits by compartmentalized partnered replication. Nature Biotechnology 32,
doi: https://doi.org/10.1242/prelights.29394
Read preprintSign up to customise the site to your preferences and to receive alerts
Register hereAlso in the bioengineering category:
Scalable and efficient generation of mouse primordial germ cell-like cells
Carly Guiltinan

Generalized Biomolecular Modeling and Design with RoseTTAFold All-Atom
Saanjbati Adhikari

Multi-pass, single-molecule nanopore reading of long protein strands with single-amino acid sensitivity
Benjamin Dominik Maier, Samantha Seah

Also in the synthetic biology category:
Enhancer cooperativity can compensate for loss of activity over large genomic distances
Milan Antonovic
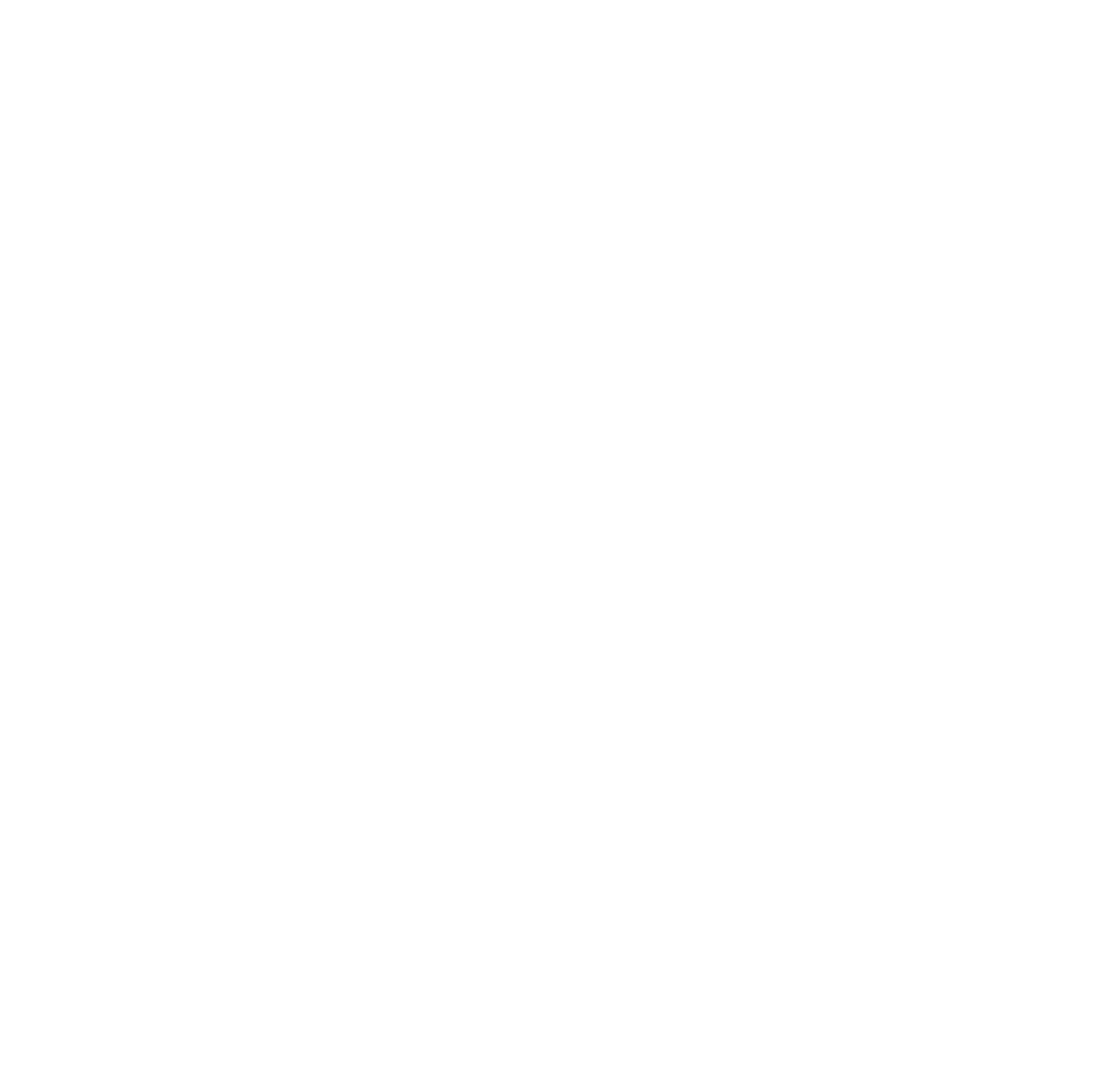
Discovery and Validation of Context-Dependent Synthetic Mammalian Promoters
Jessica L. Teo

Genetically encoded multimeric tags for intracellular protein localisation in cryo-EM
Martyna Kosno-Vega
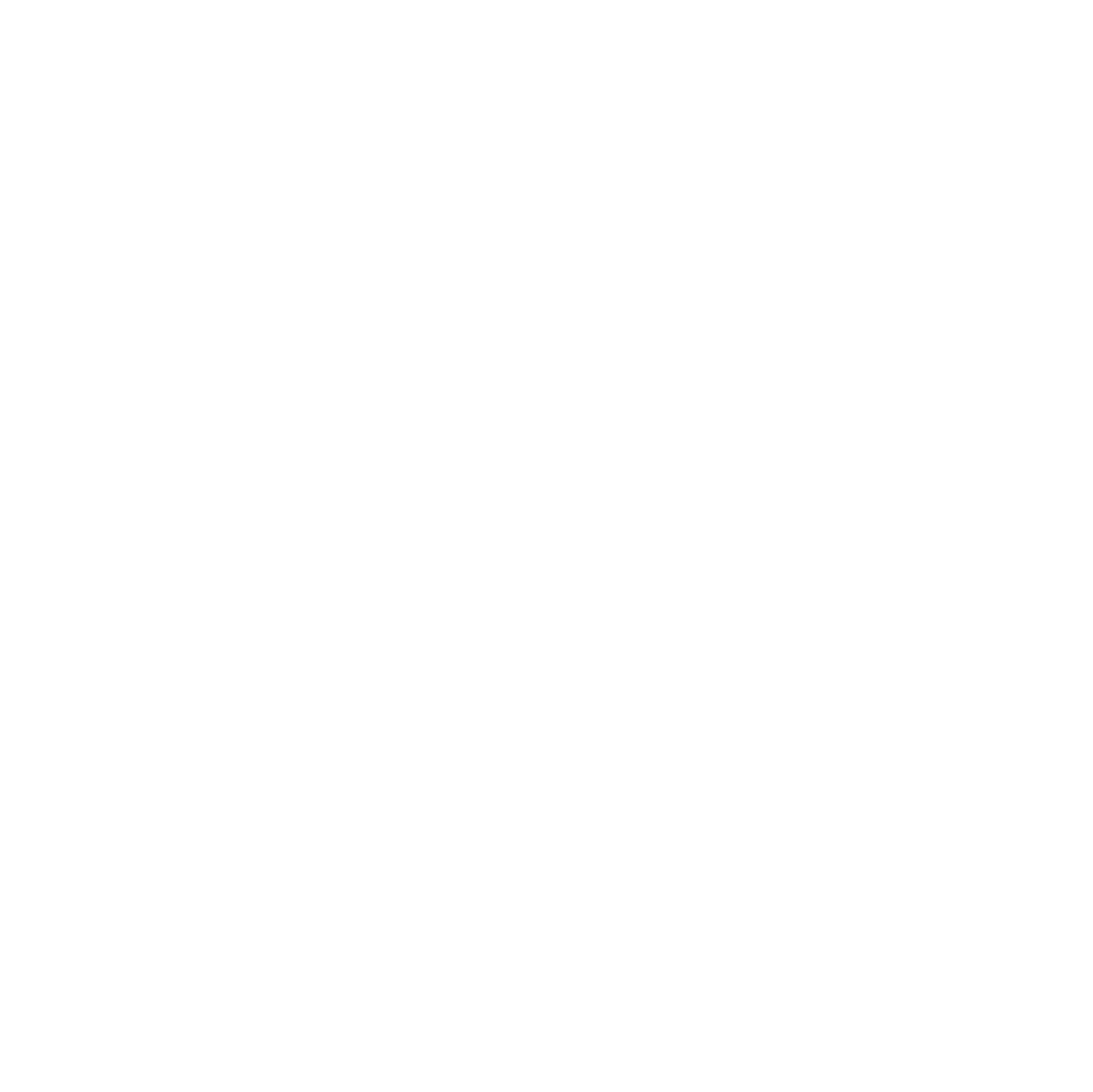
preListsbioengineering category:
in theCSHL 87th Symposium: Stem Cells
Preprints mentioned by speakers at the #CSHLsymp23
List by | Alex Eve |
EMBL Synthetic Morphogenesis: From Gene Circuits to Tissue Architecture (2021)
A list of preprints mentioned at the #EESmorphoG virtual meeting in 2021.
List by | Alex Eve |
3D Gastruloids
A curated list of preprints related to Gastruloids (in vitro models of early development obtained by 3D aggregation of embryonic cells). Updated until July 2021.
List by | Paul Gerald L. Sanchez and Stefano Vianello |
ASCB EMBO Annual Meeting 2019
A collection of preprints presented at the 2019 ASCB EMBO Meeting in Washington, DC (December 7-11)
List by | Madhuja Samaddar et al. |
EMBL Seeing is Believing – Imaging the Molecular Processes of Life
Preprints discussed at the 2019 edition of Seeing is Believing, at EMBL Heidelberg from the 9th-12th October 2019
List by | Dey Lab |
Lung Disease and Regeneration
This preprint list compiles highlights from the field of lung biology.
List by | Rob Hynds |
Advances in microscopy
This preList highlights exciting unpublished preprint articles describing advances in microscopy with a focus on light-sheet microscopy.
List by | Stephan Daetwyler |
Also in the synthetic biology category:
‘In preprints’ from Development 2022-2023
A list of the preprints featured in Development's 'In preprints' articles between 2022-2023
List by | Alex Eve, Katherine Brown |
EMBL Synthetic Morphogenesis: From Gene Circuits to Tissue Architecture (2021)
A list of preprints mentioned at the #EESmorphoG virtual meeting in 2021.
List by | Alex Eve |
EMBL Conference: From functional genomics to systems biology
Preprints presented at the virtual EMBL conference "from functional genomics and systems biology", 16-19 November 2020
List by | Jesus Victorino |
Antimicrobials: Discovery, clinical use, and development of resistance
Preprints that describe the discovery of new antimicrobials and any improvements made regarding their clinical use. Includes preprints that detail the factors affecting antimicrobial selection and the development of antimicrobial resistance.
List by | Zhang-He Goh |
Advances in Drug Delivery
Advances in formulation technology or targeted delivery methods that describe or develop the distribution of small molecules or large macromolecules to specific parts of the body.
List by | Zhang-He Goh |