Imaging mechanical properties of sub-micron ECM in live zebrafish using Brillouin microscopy
Preprint posted on 10 December 2018 https://www.biorxiv.org/content/early/2018/12/10/491803
Article now published in Biomedical Optics Express at http://dx.doi.org/10.1364/boe.10.001420
How to measure ECM in living organisms with high-resolution but without labels? A new preprint from the lab of Diz-Muñoz and @Prevedel_Lab gives an answer:
Selected by Stephan DaetwylerCategories: bioengineering, biophysics
Context
It is becoming increasingly clear that mechanical cues are important for cell survival, behavior and fate determination [1]. Our understanding of these mechanical cues, however, is far from complete due to the difficulty to measure and perturb them in a precise manner in vivo. While techniques such as atomic force microscopy [2], micropipette aspiration [3], or optical tweezers [4] have been instrumental in measuring mechanical forces, they are invasive techniques and consequently their application for in vivo measurements is limited. By using the frequency shift observed in scattered light, Brillouin microscopy offers an alternative non-invasive optical microscopy technique to measure material properties in living cells and tissues [5,6].
What is Brillouin microscopy (a short technical background)
In 1922, Léon Brillouin discovered that light is scattered by inherent density fluctuations in materials that are induced by thermally-driven collective oscillations of atoms and/or molecules (acoustic phonons). This scattering is inelastic and thereby induces a tiny frequency shift between the incident and scattered light. Brillouin microscopy measures this frequency shift. The Brillouin frequency shift has been shown to be related to the longitudinal elastic modulus (inverse compressibility) of the imaged material and its density (Eq. 1). Furthermore, the shift depends on the refractive index and parameters controlled by the microscope setup such as the angle of scattering and the light wavelength. As Brillouin microscopy relies directly on the material property of biological structures and tissues imaged, it is label-free.

Key findings and why I chose this preprint
For the first time, Brillouin microscopy has been shown to measure the thickness of ECM in vivo in the zebrafish notochord with high resolution (Fig. 1). The authors nicely compare this measurement to thickness measurements obtained with electron microscopy. Both imaging modalities agree. As Brillouin microscopy is label-free, it does not interfere with fluorescent imaging and therefore provides a complementary imaging modality to confocal or light sheet imaging. Consequently, Brillouin microscopy opens up the door for new, complex experiments.
In this paper, the authors also point out that Brillouin microscopy is subject to the challenge of many optical systems, i.e. the anisotropy of the system point spread function (PSF). Keeping this in mind is important for the interpretation of the resulting material property map.
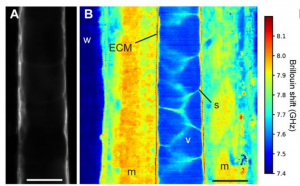
Future directions and interesting follow-up questions
- How do the measurements of ECM thickness vary over development and along the anterior-posterior axis?
- Pioneering work of determining ECM components have been done by Palombo et al. [8]. Can the authors determine from their measurements different ECM components, potentially also over development?
- There is an ongoing debate whether the longitudinal elastic modulus is important for biological processes, for example whether it is correlated to the Young’s modulus that is a measure of the stiffness of a material [6,7]. Is the Young’s modulus and thus stiffness in ECM related to the longitudinal elastic modulus?
- Currently, the exposure time is 0.25 seconds per pixel. Can the acquisition time be accelerated?
References
[1] Bloom, A. B., & Zaman, M. H. (2014). Influence of the microenvironment on cell fate determination and migration. Physiological genomics.
[2] Krieg, M., Fläschner, G., Alsteens, D., Gaub, B. M., Roos, W. H., Wuite, G. J. L., et al. (2018). Atomic force microscopy-based mechanobiology. Nature Reviews Physics.
[3] Guevorkian, K., & Maitre, J.-L. (2017). Micropipette aspiration: A unique tool for exploring cell and tissue mechanics in vivo. Methods Cell Biol.
[4] Killian, J. L., Ye, F., & Wang, M. D. (2018). Optical Tweezers: A Force to Be Reckoned With. Cell.
[5] Scarcelli, G., & Yun, S. H. (2007). Confocal Brillouin microscopy for three-dimensional mechanical imaging. Nature photonics.
[6] Schlussler, R., Mollmert, S., Abuhattum, S., Cojoc, G., Muller, P., Kim, K., et al. (2018). Mechanical Mapping of Spinal Cord Growth and Repair in Living Zebrafish Larvae by Brillouin Imaging. Biophysical Journal.
[7] Wu, P.-J., Kabakova, I. V., Ruberti, J. W., Sherwood, J. M., Dunlop, I. E., Paterson, C., et al. (2018). Water content, not stiffness, dominates Brillouin spectroscopy measurements in hydrated materials. Nature Methods.
[8] Palombo, F., Winlove, C. P., Edginton, R. S., Green, E. , Stone, N., Caponi, S., Madami, M. & Fioretto, D. (2014). Biomechanics of fibrous proteins of the extracellular matrix studied by Brillouin scattering. Journal of The Royal Society, Inteface.
Posted on: 8 January 2019 , updated on: 9 January 2019
doi: https://doi.org/10.1242/prelights.7023
Read preprintSign up to customise the site to your preferences and to receive alerts
Register hereAlso in the bioengineering category:
Scalable and efficient generation of mouse primordial germ cell-like cells
Generalized Biomolecular Modeling and Design with RoseTTAFold All-Atom
Multi-pass, single-molecule nanopore reading of long protein strands with single-amino acid sensitivity
Also in the biophysics category:
Structural basis of respiratory complexes adaptation to cold temperatures
Actin polymerization drives lumen formation in a human epiblast model
Learning a conserved mechanism for early neuroectoderm morphogenesis
preListsbioengineering category:
in theCSHL 87th Symposium: Stem Cells
Preprints mentioned by speakers at the #CSHLsymp23
List by | Alex Eve |
EMBL Synthetic Morphogenesis: From Gene Circuits to Tissue Architecture (2021)
A list of preprints mentioned at the #EESmorphoG virtual meeting in 2021.
List by | Alex Eve |
3D Gastruloids
A curated list of preprints related to Gastruloids (in vitro models of early development obtained by 3D aggregation of embryonic cells). Updated until July 2021.
List by | Paul Gerald L. Sanchez and Stefano Vianello |
ASCB EMBO Annual Meeting 2019
A collection of preprints presented at the 2019 ASCB EMBO Meeting in Washington, DC (December 7-11)
List by | Madhuja Samaddar et al. |
EMBL Seeing is Believing – Imaging the Molecular Processes of Life
Preprints discussed at the 2019 edition of Seeing is Believing, at EMBL Heidelberg from the 9th-12th October 2019
List by | Dey Lab |
Lung Disease and Regeneration
This preprint list compiles highlights from the field of lung biology.
List by | Rob Hynds |
Advances in microscopy
This preList highlights exciting unpublished preprint articles describing advances in microscopy with a focus on light-sheet microscopy.
List by | Stephan Daetwyler |
Also in the biophysics category:
preLights peer support – preprints of interest
This is a preprint repository to organise the preprints and preLights covered through the 'preLights peer support' initiative.
List by | preLights peer support |
66th Biophysical Society Annual Meeting, 2022
Preprints presented at the 66th BPS Annual Meeting, Feb 19 - 23, 2022 (The below list is not exhaustive and the preprints are listed in no particular order.)
List by | Soni Mohapatra |
EMBL Synthetic Morphogenesis: From Gene Circuits to Tissue Architecture (2021)
A list of preprints mentioned at the #EESmorphoG virtual meeting in 2021.
List by | Alex Eve |
Biophysical Society Meeting 2020
Some preprints presented at the Biophysical Society Meeting 2020 in San Diego, USA.
List by | Tessa Sinnige |
ASCB EMBO Annual Meeting 2019
A collection of preprints presented at the 2019 ASCB EMBO Meeting in Washington, DC (December 7-11)
List by | Madhuja Samaddar et al. |
EMBL Seeing is Believing – Imaging the Molecular Processes of Life
Preprints discussed at the 2019 edition of Seeing is Believing, at EMBL Heidelberg from the 9th-12th October 2019
List by | Dey Lab |
Biomolecular NMR
Preprints related to the application and development of biomolecular NMR spectroscopy
List by | Reid Alderson |
Biophysical Society Annual Meeting 2019
Few of the preprints that were discussed in the recent BPS annual meeting at Baltimore, USA
List by | Joseph Jose Thottacherry |