SF3B1-targeted Splicing Inhibition Triggers Global Alterations in Transcriptional Dynamics and R-Loop Metabolism
Posted on: 6 November 2020
Preprint posted on 8 June 2020
Debunking the interplay between RNA splicing and R-loops: Old game, new rules?
Selected by Sree Rama ChaitanyaCategories: biochemistry, bioinformatics, genomics, molecular biology
Context1-4
We all heard of single- or double-stranded DNA and RNA. But cells in all living organisms also produce hybrids of RNA and DNA (also called RNA-DNA hybrids). Short stretches of RNA-DNA hybrids are synthesized transiently during transcription and DNA replication, leading to the notion that RNA-DNA hybrids are byproducts of these important genomic processes.
During transcription, the nascent RNA synthesized within the transcription bubble comes out of the RNA Polymerase II (RNAPII) exit channel separated from the duplex DNA. Under favorable conditions, this nascent RNA can re-hybridize with its complementary DNA strand outside the transcription bubble forming a triple-helical nucleic acid structure with an RNA-DNA hybrid and a displaced single-strand of DNA called an R-loop. R-loop structures are ubiquitous and regulated by various cis– and trans-acting factors like sequence composition, DNA superhelicity, nucleosome dynamics, and co-transcriptional RNA processing. Interestingly, the past two decades of research demonstrate that R-loops are biologically relevant and regulate many genomic processes like transcription, DNA recombination and repair.
Besides orchestrating efficient RNA processing, several RNA processing factors were proposed to prevent R-loop formation. For example, RNA splicing factors were thought to prevent R-loops by sequestering nascent RNA from binding to complementary DNA strand and by physically limiting the homology between RNA and DNA through the splicing reaction. Dysregulation of one or more RNA splicing factors induced by inhibition or loss-of-function mutations was proposed to induce aberrant R-loop levels, leading to genome instability in cells. However, mechanistic insights into the aforementioned processes are not clear (mostly cause of the technical challenges involved in detecting cellular R-loops5). Therefore, the authors of the current study set out to investigate how dysregulation of RNA splicing impacts co-transcriptional R-loops.
Key findings
- To understand the impact of RNA splicing on R-loop distribution, the authors chemically inhibited the U2 spliceosome complex using Pladienolide B (PladB) in human immortalized myelogenous leukemia cells (K-562). They gauged genome-wide R-loops, nascent RNA, and steady-state RNA levels using established sequence-based approaches DRIP-seq, EU-seq, and RNA-seq, respectively. They found that PladB mediated splicing inhibition impinges on global transcription and R-loop distribution in K-562 cells.
- They demonstrate that, as expected, PladB treatment caused splicing anomalies, predominantly intron-retention, and to a lesser extent skipped-exons. Surprisingly, they found that PladB-mediated R-loop gains only correlated with 2% of genomic locales showing intron-retention. Thus, they report that loci with PladB-mediated intron-retention do not associate with increased R-loops.
- Contrary to their initial hypothesis, they found that PladB treatment significantly reduced R-loop levels genome-wide with R-loop losses outnumbering gains (i.e., 15 to 1). PladB reduced R-loops through transcribed gene bodies, with a gradual and directional loss leading to prominent effects at gene termini. Importantly, nascent RNA levels corroborated this global R-loop loss. About 77% of genes exhibiting lower nascent transcription manifested lower R-loops, reinforcing the co-transcriptional nature of R-loops. Their data reiterates earlier findings that splicing dynamics feedbacks onto transcription rates6. Moreover, genes showing R-loop loss harbored a lower pool of RNAPII paused at promoter-proximal regions (indicating that these genes lack a robust store of promoter-proximal paused RNAPII). Thus, they report that PladB-mediated R-loop losses through gene bodies result from an inhibitory effect of splicing inhibition on transcription elongation.
- They further investigated the characteristics of PladB-mediated R-loop gains. About two-thirds of PladB-mediated R-loop gains mapped to intergenic regions, extending downstream of RNAPII transcribing genes present in gene-sparse neighborhoods. These new R-loop zones were ~50kb long and again correlated with nascent RNA profiles that manifested a readthrough transcription after the poly-A signals (PAS). Furthermore, PladB treatment exaggerated the pausing signature of RNAPII at promoter-proximal regions followed by deficiencies in RNAPII elongation and distal splicing events at 3’ end of these genes. Thus, PladB treatment seems to alter RNAPII regulation and propel it to readthrough towards downstream intergenic regions post-PAS (in a subset of ~300 genes).
- Previous findings suggest that defects in splicing proteins induce aberrant R-loop levels that cause DNA damage. However, the investigators found that PladB treatment only induced γH2AX (DNA damage marker) levels marginally, even at longer time points (24 hrs). Furthermore, they did not find any significant impact of PladB-mediated R-loops on γH2AX levels at gene loci (assessed by ChIP, chromatin immunoprecipitation). Additionally, they also found that PladB treatment reduced the expression of genes involved in DNA damage response pathways. Thus, they suggest that splicing inhibition via PladB might not directly cause R-loop mediated DNA damage.
Schematic representation of the effects of pre-mRNA splicing on the genomic distribution of R-loops. Taken and modified directly from Castillo-Guzman D et. al., 2020 under a CC-BY 4.0 international license.
Perspective
The past two decades of research expound on the design principles of R-loop formation and dynamics. The consensus is that dysregulation of RNA processing factors (like splicing factors) leads to aberrant R-loops that cause genome instability, a hallmark of cancer. Moreover, targeting splicing machinery surfaced as an important strategy to alleviate cancer7. Therefore, understanding the mechanistic insights of R-loop regulation could support combinatorial therapeutic strategies to mitigate cancer progression.
Acknowledgments
I am thankful to all the authors for their support, especially Frédéric Chédin for taking the time in an often busy schedule to comment on the preLight.
References
- https://doi.org/10.1016/j.cell.2019.08.055
- https://doi.org/10.1038/s41580-019-0206-3
- https://dx.doi.org/10.1016%2Fj.tig.2016.10.002
- https://doi.org/10.1016/j.molcel.2019.01.024
- https://doi.org/10.1242/prelights.21214
- https://doi.org/10.1038/nsmb.2124
- https://doi.org/10.1038/s41573-019-0042-3
- http://dx.doi.org/10.1016/j.molcel.2017.06.027
- https://dx.doi.org/10.1016%2Fj.molcel.2017.10.008
- https://doi.org/10.1016/j.jmb.2020.02.014
doi: https://doi.org/10.1242/prelights.25281
Read preprintSign up to customise the site to your preferences and to receive alerts
Register hereAlso in the biochemistry category:
Notch3 is a genetic modifier of NODAL signalling for patterning asymmetry during mouse heart looping
Bhaval Parmar
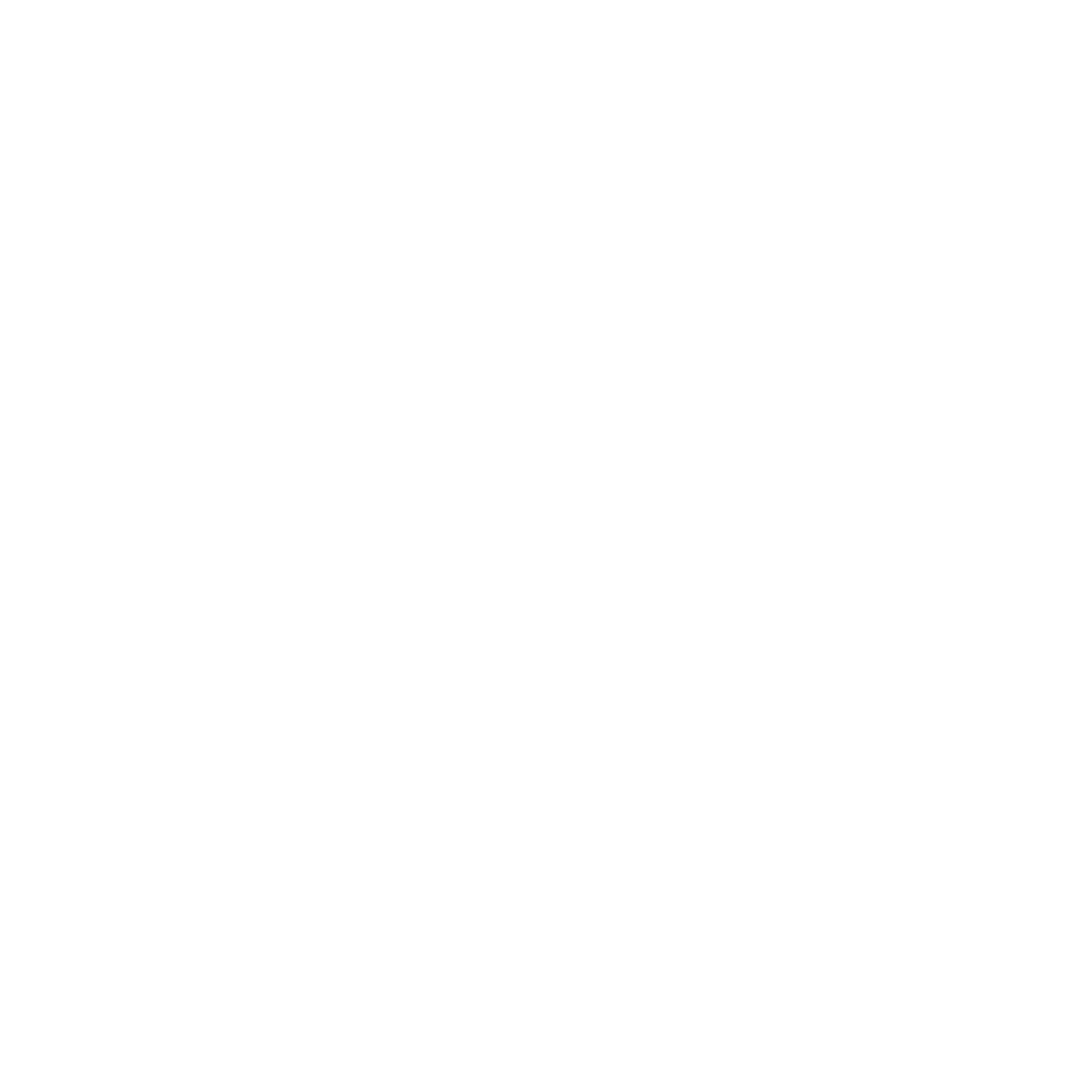
14-3-3 binding regulates Tau assembly and microtubule association
Barbora Knotkova et al.
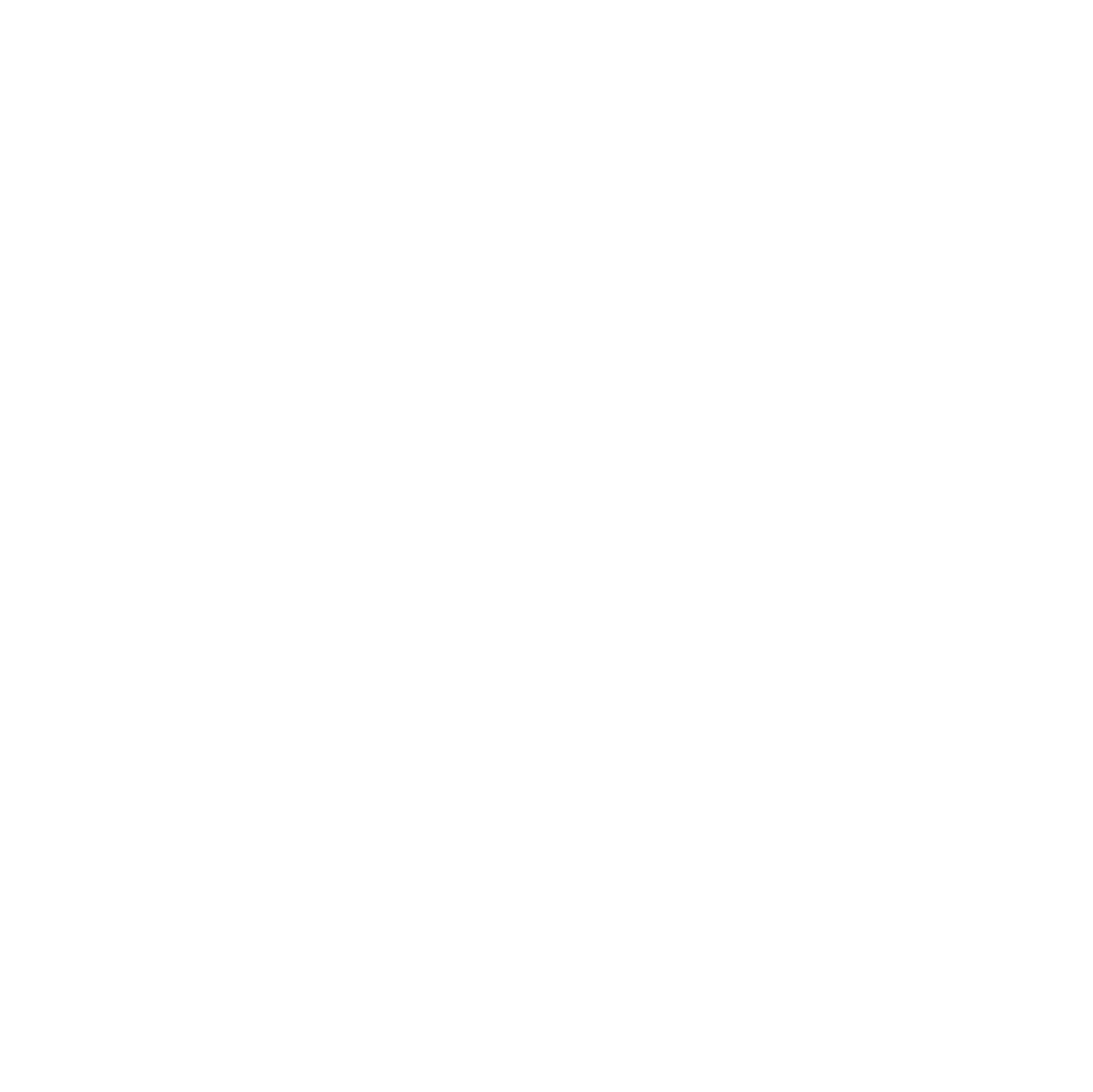
Structural basis of respiratory complexes adaptation to cold temperatures
Pamela Ornelas
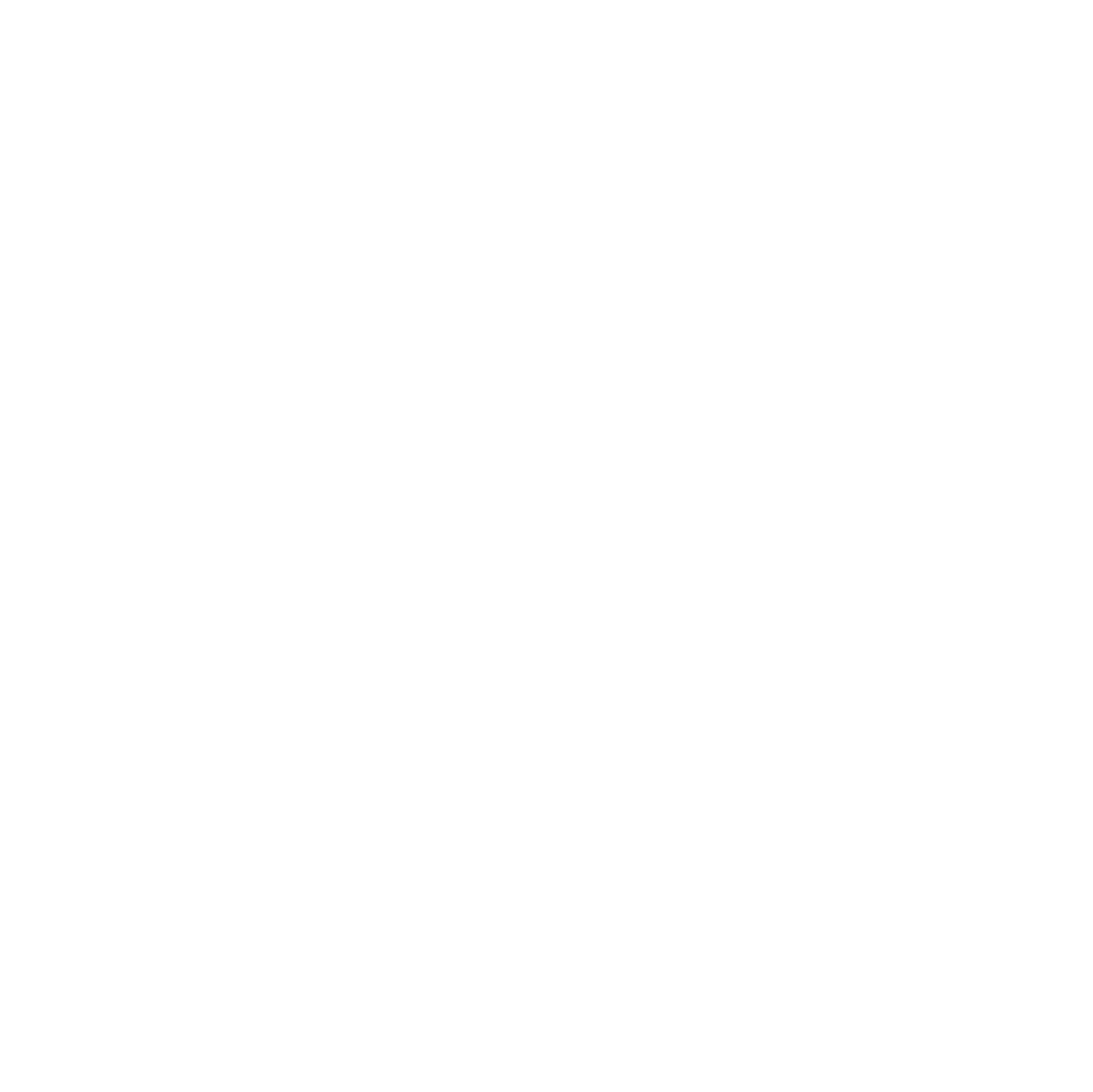
Also in the bioinformatics category:
Enhancer-driven cell type comparison reveals similarities between the mammalian and bird pallium
Rodrigo Senovilla-Ganzo

Expressive modeling and fast simulation for dynamic compartments
Benjamin Dominik Maier
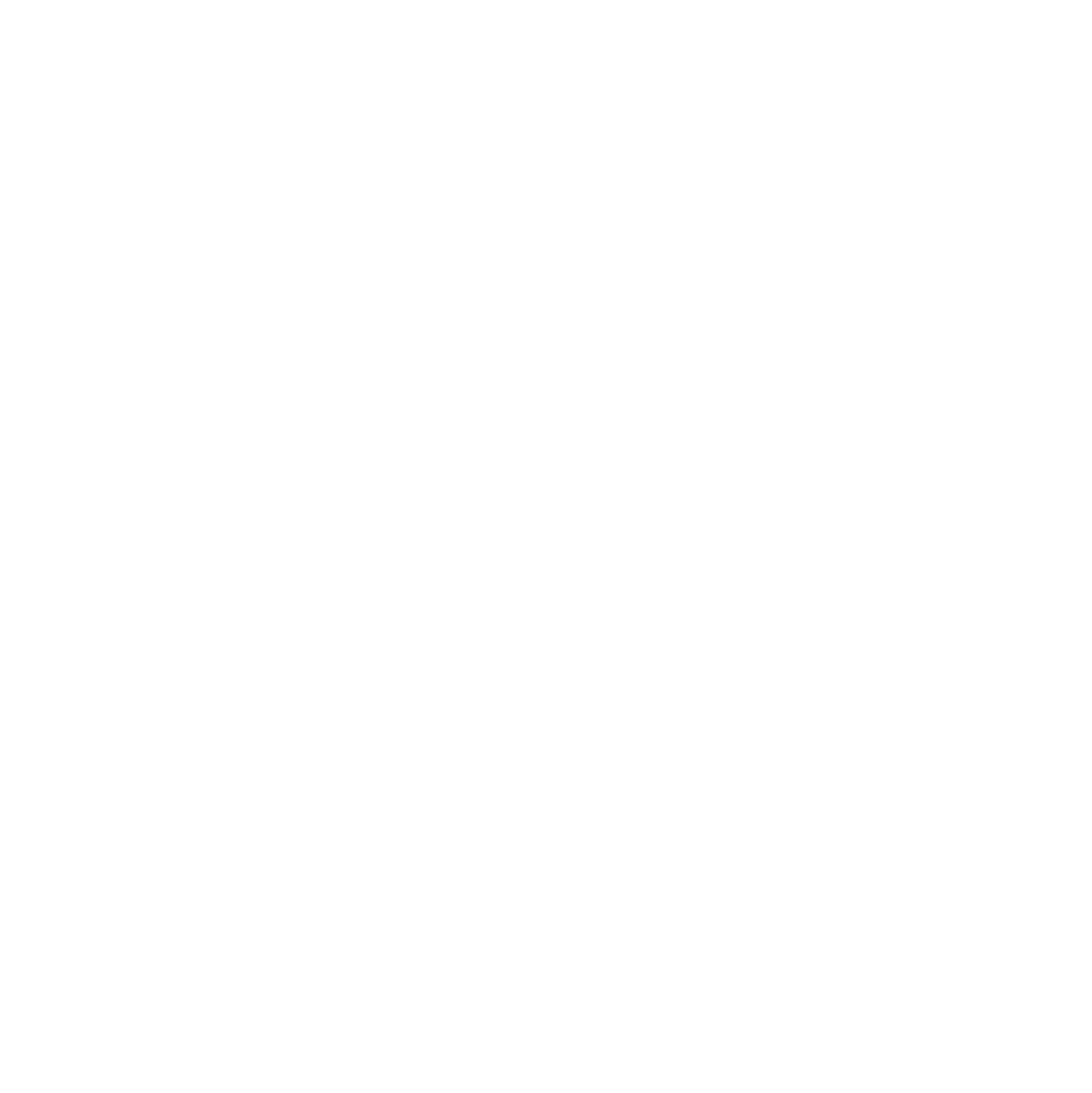
Transcriptional profiling of human brain cortex identifies novel lncRNA-mediated networks dysregulated in amyotrophic lateral sclerosis
Julio Molina Pineda
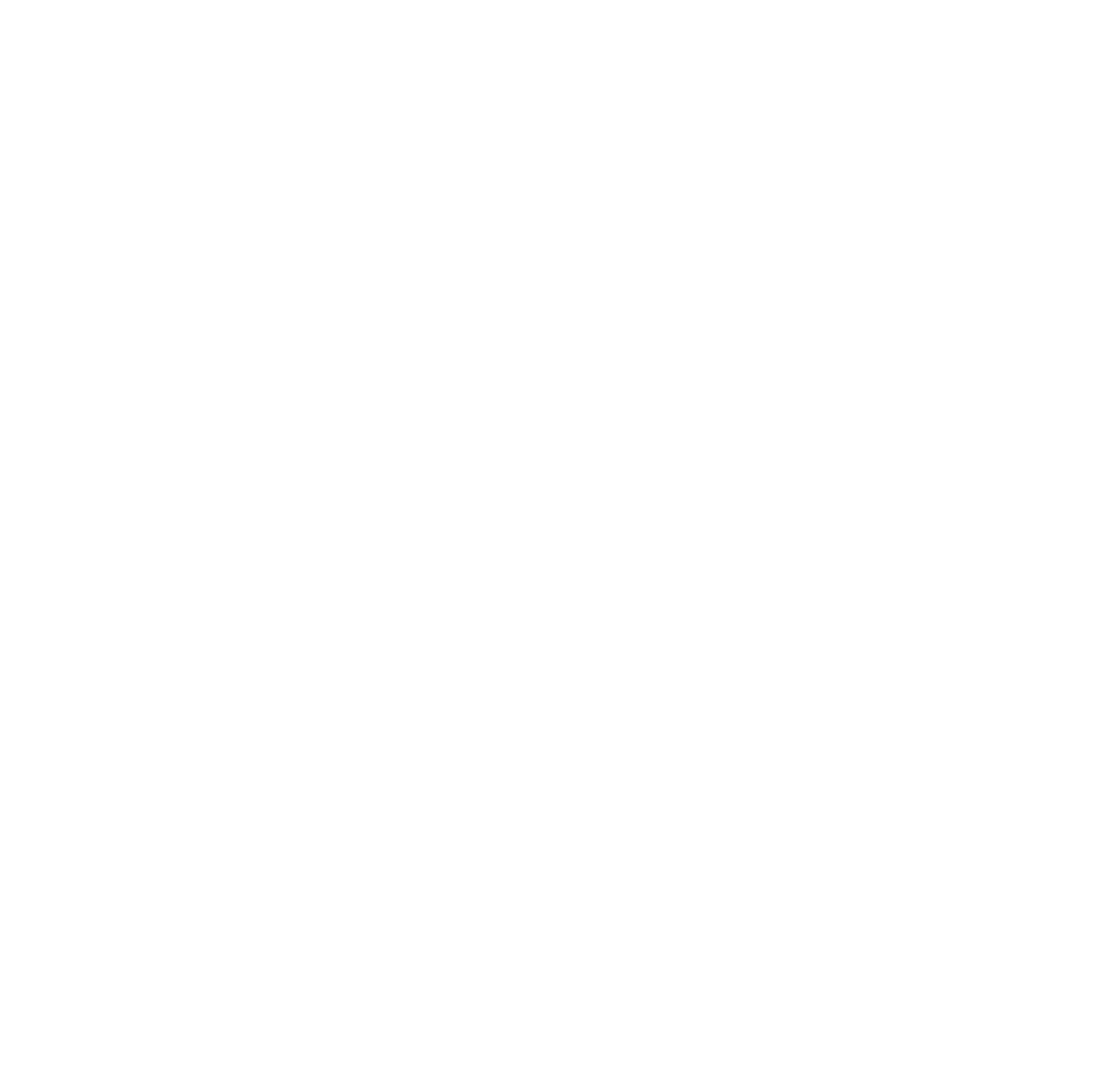
Also in the genomics category:
A fine kinetic balance of interactions directs transcription factor hubs to genes
Deevitha Balasubramanian
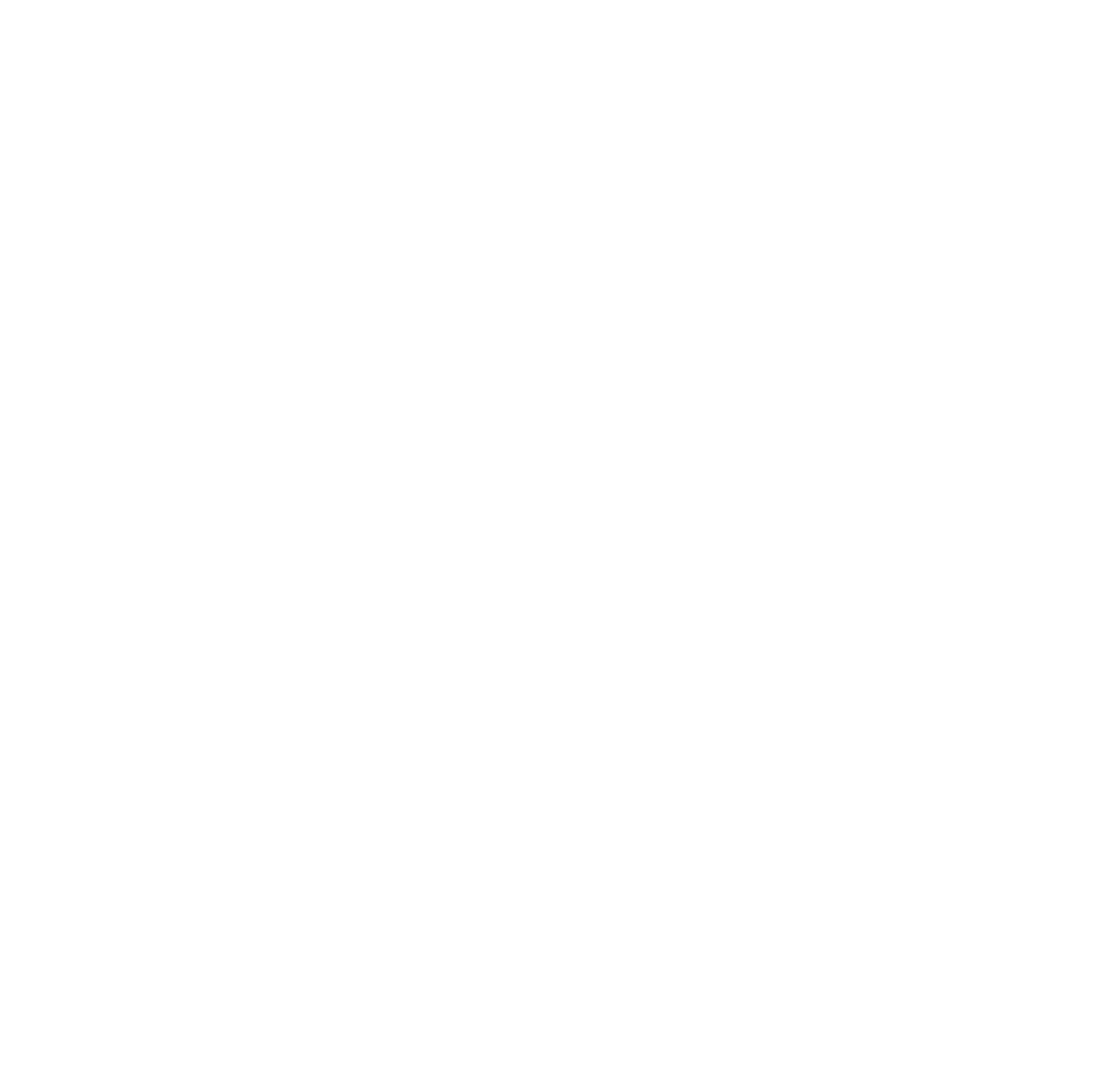
Enhancer-driven cell type comparison reveals similarities between the mammalian and bird pallium
Rodrigo Senovilla-Ganzo

Modular control of time and space during vertebrate axis segmentation
AND
Natural genetic variation quantitatively regulates heart rate and dimension
Girish Kale, Jennifer Ann Black
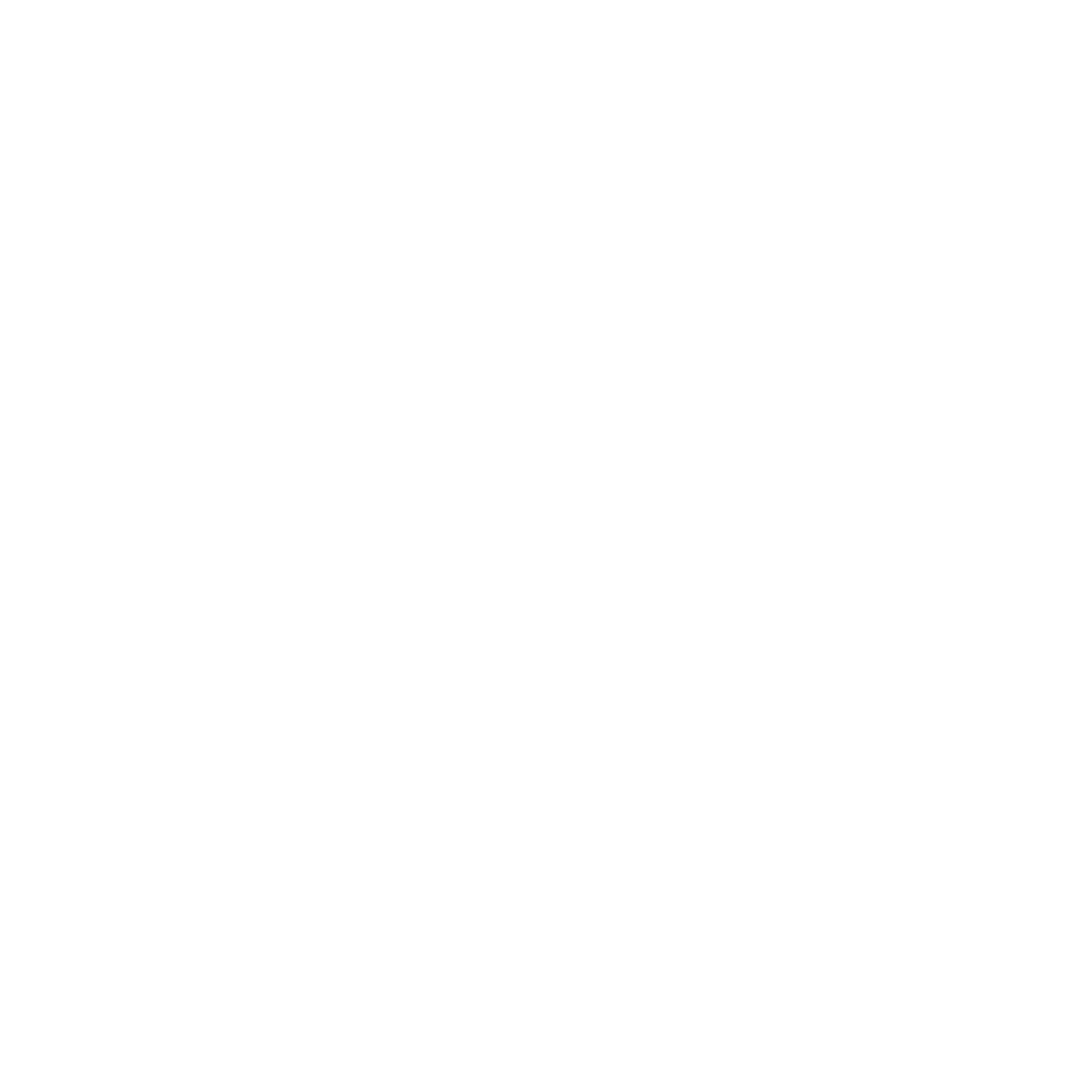
Also in the molecular biology category:
Cell cycle-dependent mRNA localization in P-bodies
Mohammed JALLOH

Notch3 is a genetic modifier of NODAL signalling for patterning asymmetry during mouse heart looping
Bhaval Parmar
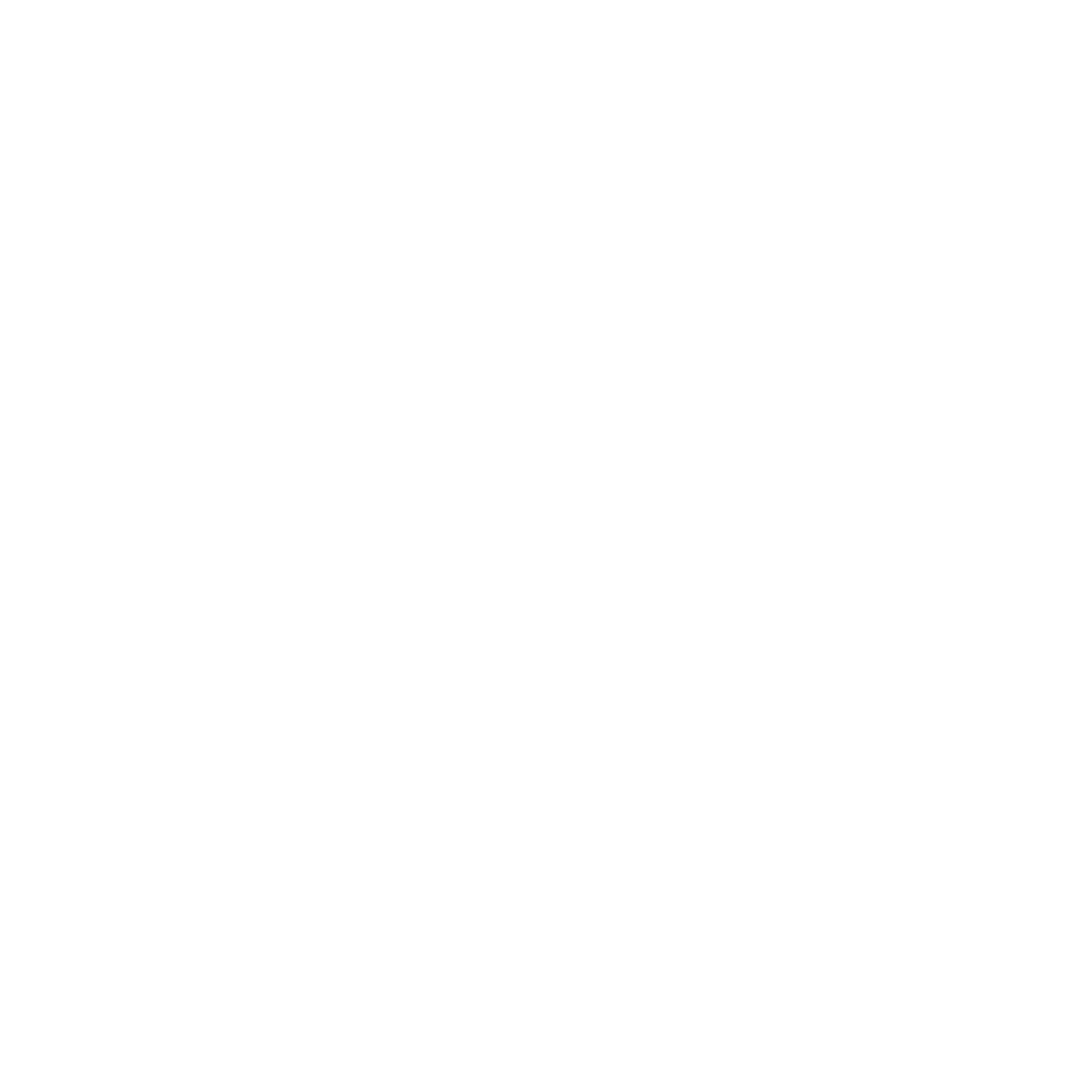
Fetal brain response to maternal inflammation requires microglia
Manuel Lessi

preListsbiochemistry category:
in theBSCB-Biochemical Society 2024 Cell Migration meeting
This preList features preprints that were discussed and presented during the BSCB-Biochemical Society 2024 Cell Migration meeting in Birmingham, UK in April 2024. Kindly put together by Sara Morais da Silva, Reviews Editor at Journal of Cell Science.
List by | Reinier Prosee |
Peer Review in Biomedical Sciences
Communication of scientific knowledge has changed dramatically in recent decades and the public perception of scientific discoveries depends on the peer review process of articles published in scientific journals. Preprints are key vehicles for the dissemination of scientific discoveries, but they are still not properly recognized by the scientific community since peer review is very limited. On the other hand, peer review is very heterogeneous and a fundamental aspect to improve it is to train young scientists on how to think critically and how to evaluate scientific knowledge in a professional way. Thus, this course aims to: i) train students on how to perform peer review of scientific manuscripts in a professional manner; ii) develop students' critical thinking; iii) contribute to the appreciation of preprints as important vehicles for the dissemination of scientific knowledge without restrictions; iv) contribute to the development of students' curricula, as their opinions will be published and indexed on the preLights platform. The evaluations will be based on qualitative analyses of the oral presentations of preprints in the field of biomedical sciences deposited in the bioRxiv server, of the critical reports written by the students, as well as of the participation of the students during the preprints discussions.
List by | Marcus Oliveira et al. |
CellBio 2022 – An ASCB/EMBO Meeting
This preLists features preprints that were discussed and presented during the CellBio 2022 meeting in Washington, DC in December 2022.
List by | Nadja Hümpfer et al. |
20th “Genetics Workshops in Hungary”, Szeged (25th, September)
In this annual conference, Hungarian geneticists, biochemists and biotechnologists presented their works. Link: http://group.szbk.u-szeged.hu/minikonf/archive/prg2021.pdf
List by | Nándor Lipták |
Fibroblasts
The advances in fibroblast biology preList explores the recent discoveries and preprints of the fibroblast world. Get ready to immerse yourself with this list created for fibroblasts aficionados and lovers, and beyond. Here, my goal is to include preprints of fibroblast biology, heterogeneity, fate, extracellular matrix, behavior, topography, single-cell atlases, spatial transcriptomics, and their matrix!
List by | Osvaldo Contreras |
ASCB EMBO Annual Meeting 2019
A collection of preprints presented at the 2019 ASCB EMBO Meeting in Washington, DC (December 7-11)
List by | Madhuja Samaddar et al. |
EMBL Seeing is Believing – Imaging the Molecular Processes of Life
Preprints discussed at the 2019 edition of Seeing is Believing, at EMBL Heidelberg from the 9th-12th October 2019
List by | Dey Lab |
Cellular metabolism
A curated list of preprints related to cellular metabolism at Biorxiv by Pablo Ranea Robles from the Prelights community. Special interest on lipid metabolism, peroxisomes and mitochondria.
List by | Pablo Ranea Robles |
MitoList
This list of preprints is focused on work expanding our knowledge on mitochondria in any organism, tissue or cell type, from the normal biology to the pathology.
List by | Sandra Franco Iborra |
Also in the bioinformatics category:
‘In preprints’ from Development 2022-2023
A list of the preprints featured in Development's 'In preprints' articles between 2022-2023
List by | Alex Eve, Katherine Brown |
9th International Symposium on the Biology of Vertebrate Sex Determination
This preList contains preprints discussed during the 9th International Symposium on the Biology of Vertebrate Sex Determination. This conference was held in Kona, Hawaii from April 17th to 21st 2023.
List by | Martin Estermann |
Alumni picks – preLights 5th Birthday
This preList contains preprints that were picked and highlighted by preLights Alumni - an initiative that was set up to mark preLights 5th birthday. More entries will follow throughout February and March 2023.
List by | Sergio Menchero et al. |
Fibroblasts
The advances in fibroblast biology preList explores the recent discoveries and preprints of the fibroblast world. Get ready to immerse yourself with this list created for fibroblasts aficionados and lovers, and beyond. Here, my goal is to include preprints of fibroblast biology, heterogeneity, fate, extracellular matrix, behavior, topography, single-cell atlases, spatial transcriptomics, and their matrix!
List by | Osvaldo Contreras |
Single Cell Biology 2020
A list of preprints mentioned at the Wellcome Genome Campus Single Cell Biology 2020 meeting.
List by | Alex Eve |
Antimicrobials: Discovery, clinical use, and development of resistance
Preprints that describe the discovery of new antimicrobials and any improvements made regarding their clinical use. Includes preprints that detail the factors affecting antimicrobial selection and the development of antimicrobial resistance.
List by | Zhang-He Goh |
Also in the genomics category:
BSCB-Biochemical Society 2024 Cell Migration meeting
This preList features preprints that were discussed and presented during the BSCB-Biochemical Society 2024 Cell Migration meeting in Birmingham, UK in April 2024. Kindly put together by Sara Morais da Silva, Reviews Editor at Journal of Cell Science.
List by | Reinier Prosee |
9th International Symposium on the Biology of Vertebrate Sex Determination
This preList contains preprints discussed during the 9th International Symposium on the Biology of Vertebrate Sex Determination. This conference was held in Kona, Hawaii from April 17th to 21st 2023.
List by | Martin Estermann |
Semmelweis Symposium 2022: 40th anniversary of international medical education at Semmelweis University
This preList contains preprints discussed during the 'Semmelweis Symposium 2022' (7-9 November), organised around the 40th anniversary of international medical education at Semmelweis University covering a wide range of topics.
List by | Nándor Lipták |
20th “Genetics Workshops in Hungary”, Szeged (25th, September)
In this annual conference, Hungarian geneticists, biochemists and biotechnologists presented their works. Link: http://group.szbk.u-szeged.hu/minikonf/archive/prg2021.pdf
List by | Nándor Lipták |
EMBL Conference: From functional genomics to systems biology
Preprints presented at the virtual EMBL conference "from functional genomics and systems biology", 16-19 November 2020
List by | Jesus Victorino |
TAGC 2020
Preprints recently presented at the virtual Allied Genetics Conference, April 22-26, 2020. #TAGC20
List by | Maiko Kitaoka et al. |
Zebrafish immunology
A compilation of cutting-edge research that uses the zebrafish as a model system to elucidate novel immunological mechanisms in health and disease.
List by | Shikha Nayar |
Also in the molecular biology category:
BSCB-Biochemical Society 2024 Cell Migration meeting
This preList features preprints that were discussed and presented during the BSCB-Biochemical Society 2024 Cell Migration meeting in Birmingham, UK in April 2024. Kindly put together by Sara Morais da Silva, Reviews Editor at Journal of Cell Science.
List by | Reinier Prosee |
‘In preprints’ from Development 2022-2023
A list of the preprints featured in Development's 'In preprints' articles between 2022-2023
List by | Alex Eve, Katherine Brown |
CSHL 87th Symposium: Stem Cells
Preprints mentioned by speakers at the #CSHLsymp23
List by | Alex Eve |
9th International Symposium on the Biology of Vertebrate Sex Determination
This preList contains preprints discussed during the 9th International Symposium on the Biology of Vertebrate Sex Determination. This conference was held in Kona, Hawaii from April 17th to 21st 2023.
List by | Martin Estermann |
Alumni picks – preLights 5th Birthday
This preList contains preprints that were picked and highlighted by preLights Alumni - an initiative that was set up to mark preLights 5th birthday. More entries will follow throughout February and March 2023.
List by | Sergio Menchero et al. |
CellBio 2022 – An ASCB/EMBO Meeting
This preLists features preprints that were discussed and presented during the CellBio 2022 meeting in Washington, DC in December 2022.
List by | Nadja Hümpfer et al. |
EMBL Synthetic Morphogenesis: From Gene Circuits to Tissue Architecture (2021)
A list of preprints mentioned at the #EESmorphoG virtual meeting in 2021.
List by | Alex Eve |
FENS 2020
A collection of preprints presented during the virtual meeting of the Federation of European Neuroscience Societies (FENS) in 2020
List by | Ana Dorrego-Rivas |
ECFG15 – Fungal biology
Preprints presented at 15th European Conference on Fungal Genetics 17-20 February 2020 Rome
List by | Hiral Shah |
ASCB EMBO Annual Meeting 2019
A collection of preprints presented at the 2019 ASCB EMBO Meeting in Washington, DC (December 7-11)
List by | Madhuja Samaddar et al. |
Lung Disease and Regeneration
This preprint list compiles highlights from the field of lung biology.
List by | Rob Hynds |
MitoList
This list of preprints is focused on work expanding our knowledge on mitochondria in any organism, tissue or cell type, from the normal biology to the pathology.
List by | Sandra Franco Iborra |