Single cell sequencing of the small and AT-skewed genome of malaria parasites
Posted on: 10 December 2020 , updated on: 23 December 2020
Preprint posted on 21 June 2020
Article now published in Genome Medicine at http://dx.doi.org/10.1186/s13073-021-00889-9
Categories: genetics, molecular biology
Background
Anti-malarial drug efficacy is threatened by the frequent emergence of resistant populations. One of the major sources of genomic variation in P. falciparum that contribute to antimalarial resistance are copy number variations (CNVs), or the amplification and deletion of a genomic region. Namely, a high rate of CNVs may initiate genomic changes that contribute to the rapid adaptation of an organism. Despite the importance of CNVs, relatively little is known about them. The majority of CNVs in P. falciparum have been identified by analysing bulk DNA, whereby the CNVs are present in a large fraction of individual parasites in the population. However, it is likely that many CNVs remain undetected because of their presence at low frequency. Although various methods have improved the detection of low frequency CNVs, they still don’t offer complete CNV detection. Recently, single cell analysis to detect low frequency CNVs within heterogeneous populations has proven to offer advantages in the detection of rare genetic variants that might be overlooked in average populations. Nevertheless, short read sequencing requires much more genomic material for library construction than the genomic content of individual Plasmodium cells. This means whole genome amplification (WGA) is required to generate sufficient DNA quantities. However, most techniques are designed for mammalian cells. To date, the detection of CNVs in single P. falciparum parasites using whole genome sequencing has not been achieved, and the application of existing WGA methods has been hindered by the parasite’s small genome size and imbalanced base composition. Recognizing that an effective P. falciparum WGA method must be highly sensitive and able to handle imbalanced base composition, Liu et al (1) present here a single cell sequencing pipeline which includes efficient isolation of single infected erythrocytes, an optimized WGA step inspired by a technique called “multiple annealing and looping-based amplification cycling” (MALBAC), and a sensitive method of assessing sample quality prior to sequencing.
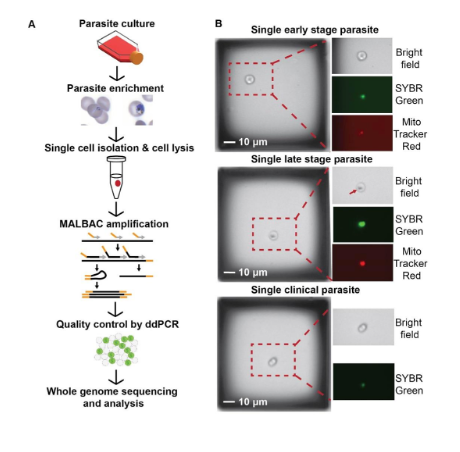
Key findings and developments
The authors began by determining whether Plasmodium falciparum genomes from single-infected erythrocytes could be amplified by MALBAC. The sequencing pipeline included stage-specific parasite enrichment, isolation of single infected erythrocytes, cell lysis, whole genome amplification, pre-sequencing quality control, whole genome sequencing, and analysis steps. For this pipeline, the authors used both a laboratory line (Dd2), or a clinical sample. For single cell isolation, they used the microscopy-based CellRaft Air system, which has the benefit of low capture volume and visual confirmation of parasite stages. On isolated samples they then applied either the standard MALBAC protocol, or an alternative version optimized for the small AT-rich P. falciparum genome. This latter method allowed amplification of 43% of the early and 90% of the late stage laboratory samples, and 100% of the clinical samples. The authors then used droplet digital PCR (ddPCR) to assess the quality of WGA products from single cells, and together with a ‘uniformity score’, then selected the genomes that had been more evenly amplified. Samples amplified with the optimized MALBAC protocol were more evenly covered than those using the standard protocol. Altogether, the authors confirmed the validity of using ddPCR detection as a quality control step prior to sequencing.
The authors showed that the optimized MALBAC method limits contamination of single cell samples. They first showed that in the clinical bulk DNA, human contamination was higher than in the laboratory Dd2 bulk DNA, as expected. The second most common source of contamination was of bacterial origin. The optimized MALBAC protocol reduced the amplification bias towards contaminating human and bacterial genomes.
As a next step, as GC-bias during the amplification step can limit which areas of the genome are sequenced, the authors evaluated whether the optimized MALBAC improved genome coverage. The authors evaluated GC bias at various steps of the pipeline, and found that the optimized MALBAC reduces amplification bias of single cell samples. The authors discuss that although the optimized MALBAC showed less bias towards GC-rich sequences, it was still problematic for highly AT-rich and repetitive intergenic regions.
Next, the authors went on to explore the uniformity of read abundance distributed over the P. falciparum genome, and found that there was improvement in levels of read uniformity across the genome when using optimized MALBAC over the standard protocol. Furthermore, the optimized MALBAC protocol was found to exhibit reproducible coverage of single cell genomes.
The authors then compared the results obtained by the optimized MALBAC protocol and an MDA-based study – which has been the only other method used to amplify single Plasmodium genomes. While MALBAC-amplified genomes exhibited a consistent amplification pattern, the MDA-amplified genomes showed substantially more variation across cells. Moreover, the correlation between MDA-amplified cells was much lower than that between the optimized MALBAC-amplified cells. However, MDA-amplified samples displayed a higher coverage breadth across the genome, especially in the intergenic regions. The authors conclude altogether than the main benefit of MALBAC over MDA-based amplification of single cell genomes is reproducible coverage with low variation.
Finally, the authors were able to perform CNV analysis in MALBAC-amplified single cell genomes through the combination of discordant/split reads and read depth analysis methods. They also further explored parameters that impacted their detection. They found that different methods exhibited differences in the ability to identify true CNVs, and they attribute this to factors including CNV size, genomic neighbourhood, and sequencing depth. In general, most of the CNVs detected by both discordant/split read and read depth analyses were spread across all but one chromosome.
The authors conclude that building on these methodological improvements will enable detection of parasite-to-parasite heterogeneity to clarify the role of genetic variations in the adaptation of P. falciparum.
What I like about this preprint
This work was presented at the Woods Hole MPM virtual meeting in 2020, and was well received by the parasitology community. I like the preprint because it aims at bridging existing methodological gaps to understand single cell heterogeneity among parasites – a topic with current great momentum in the parasitology community. It specifically addresses complications faced in the P. falciparum AT-rich genome, and could open further opportunities for single cell analysis in this and other parasites.
References
- Liu et al, Single cell sequencing of the small and AT-skewed genome of malaria parasites, bioRxiv, 2020.
doi: https://doi.org/10.1242/prelights.26295
Read preprintSign up to customise the site to your preferences and to receive alerts
Register hereAlso in the genetics category:
Enhancer-driven cell type comparison reveals similarities between the mammalian and bird pallium
Rodrigo Senovilla-Ganzo

Lipid-Based Transfection of Zebrafish Embryos: A Robust Protocol for Nucleic Acid Delivery
Roberto Rodríguez-Morales
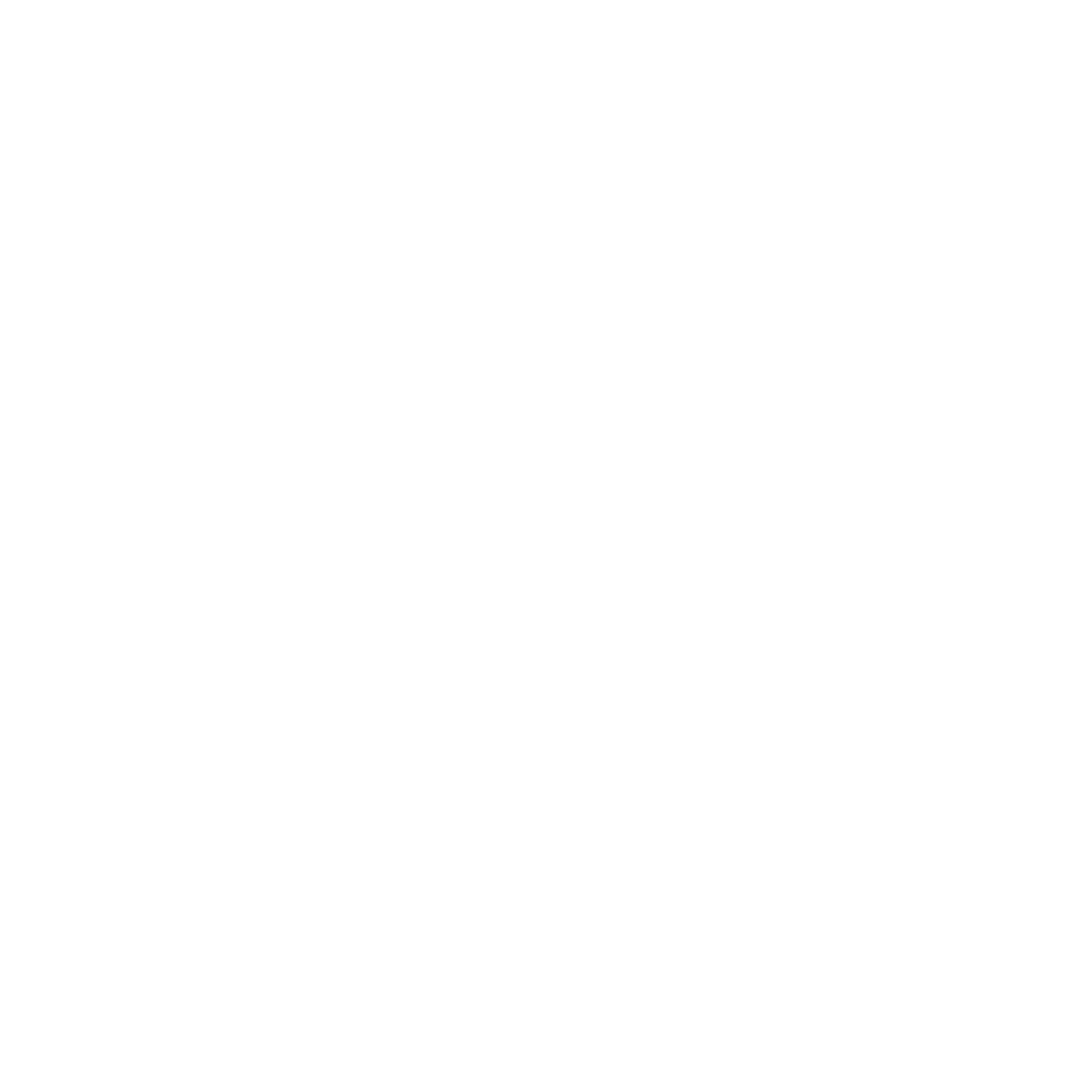
Temporal constraints on enhancer usage shape the regulation of limb gene transcription
María Mariner-Faulí
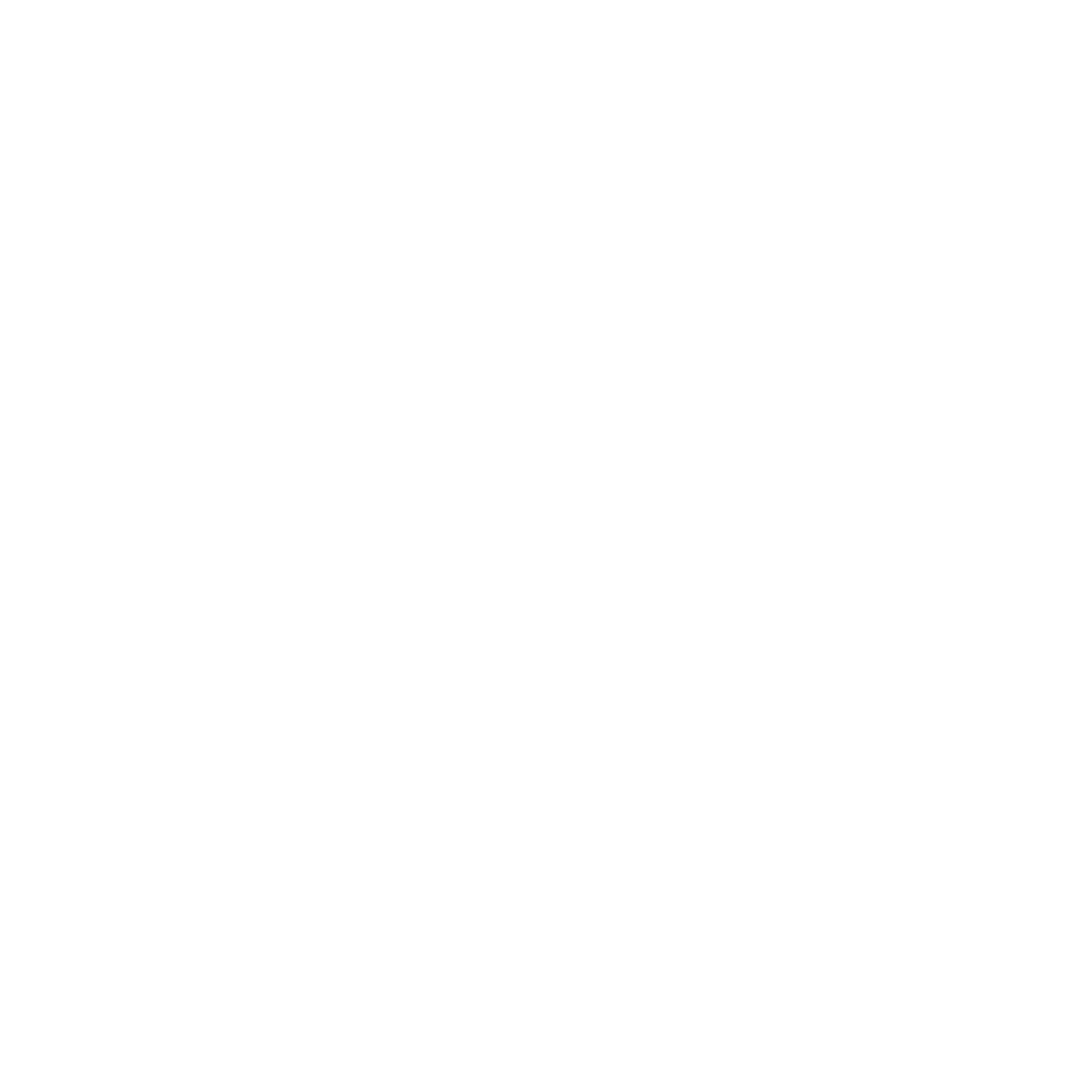
Also in the molecular biology category:
Cell cycle-dependent mRNA localization in P-bodies
Mohammed JALLOH

Notch3 is a genetic modifier of NODAL signalling for patterning asymmetry during mouse heart looping
Bhaval Parmar
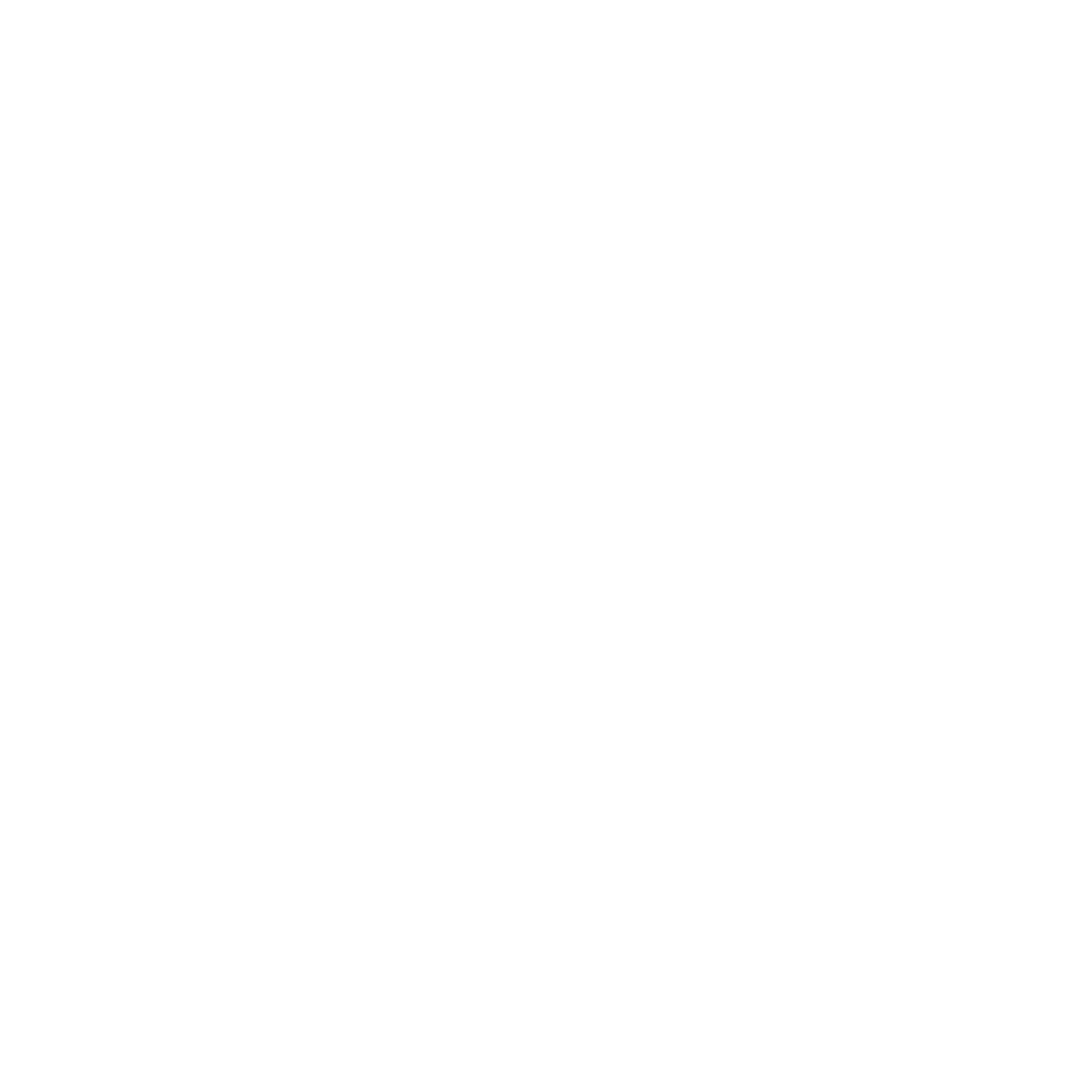
Fetal brain response to maternal inflammation requires microglia
Manuel Lessi

preListsgenetics category:
in theBSDB/GenSoc Spring Meeting 2024
A list of preprints highlighted at the British Society for Developmental Biology and Genetics Society joint Spring meeting 2024 at Warwick, UK.
List by | Joyce Yu, Katherine Brown |
BSCB-Biochemical Society 2024 Cell Migration meeting
This preList features preprints that were discussed and presented during the BSCB-Biochemical Society 2024 Cell Migration meeting in Birmingham, UK in April 2024. Kindly put together by Sara Morais da Silva, Reviews Editor at Journal of Cell Science.
List by | Reinier Prosee |
9th International Symposium on the Biology of Vertebrate Sex Determination
This preList contains preprints discussed during the 9th International Symposium on the Biology of Vertebrate Sex Determination. This conference was held in Kona, Hawaii from April 17th to 21st 2023.
List by | Martin Estermann |
Alumni picks – preLights 5th Birthday
This preList contains preprints that were picked and highlighted by preLights Alumni - an initiative that was set up to mark preLights 5th birthday. More entries will follow throughout February and March 2023.
List by | Sergio Menchero et al. |
Semmelweis Symposium 2022: 40th anniversary of international medical education at Semmelweis University
This preList contains preprints discussed during the 'Semmelweis Symposium 2022' (7-9 November), organised around the 40th anniversary of international medical education at Semmelweis University covering a wide range of topics.
List by | Nándor Lipták |
20th “Genetics Workshops in Hungary”, Szeged (25th, September)
In this annual conference, Hungarian geneticists, biochemists and biotechnologists presented their works. Link: http://group.szbk.u-szeged.hu/minikonf/archive/prg2021.pdf
List by | Nándor Lipták |
2nd Conference of the Visegrád Group Society for Developmental Biology
Preprints from the 2nd Conference of the Visegrád Group Society for Developmental Biology (2-5 September, 2021, Szeged, Hungary)
List by | Nándor Lipták |
EMBL Conference: From functional genomics to systems biology
Preprints presented at the virtual EMBL conference "from functional genomics and systems biology", 16-19 November 2020
List by | Jesus Victorino |
TAGC 2020
Preprints recently presented at the virtual Allied Genetics Conference, April 22-26, 2020. #TAGC20
List by | Maiko Kitaoka et al. |
ECFG15 – Fungal biology
Preprints presented at 15th European Conference on Fungal Genetics 17-20 February 2020 Rome
List by | Hiral Shah |
Autophagy
Preprints on autophagy and lysosomal degradation and its role in neurodegeneration and disease. Includes molecular mechanisms, upstream signalling and regulation as well as studies on pharmaceutical interventions to upregulate the process.
List by | Sandra Malmgren Hill |
Zebrafish immunology
A compilation of cutting-edge research that uses the zebrafish as a model system to elucidate novel immunological mechanisms in health and disease.
List by | Shikha Nayar |
Also in the molecular biology category:
BSCB-Biochemical Society 2024 Cell Migration meeting
This preList features preprints that were discussed and presented during the BSCB-Biochemical Society 2024 Cell Migration meeting in Birmingham, UK in April 2024. Kindly put together by Sara Morais da Silva, Reviews Editor at Journal of Cell Science.
List by | Reinier Prosee |
‘In preprints’ from Development 2022-2023
A list of the preprints featured in Development's 'In preprints' articles between 2022-2023
List by | Alex Eve, Katherine Brown |
CSHL 87th Symposium: Stem Cells
Preprints mentioned by speakers at the #CSHLsymp23
List by | Alex Eve |
9th International Symposium on the Biology of Vertebrate Sex Determination
This preList contains preprints discussed during the 9th International Symposium on the Biology of Vertebrate Sex Determination. This conference was held in Kona, Hawaii from April 17th to 21st 2023.
List by | Martin Estermann |
Alumni picks – preLights 5th Birthday
This preList contains preprints that were picked and highlighted by preLights Alumni - an initiative that was set up to mark preLights 5th birthday. More entries will follow throughout February and March 2023.
List by | Sergio Menchero et al. |
CellBio 2022 – An ASCB/EMBO Meeting
This preLists features preprints that were discussed and presented during the CellBio 2022 meeting in Washington, DC in December 2022.
List by | Nadja Hümpfer et al. |
EMBL Synthetic Morphogenesis: From Gene Circuits to Tissue Architecture (2021)
A list of preprints mentioned at the #EESmorphoG virtual meeting in 2021.
List by | Alex Eve |
FENS 2020
A collection of preprints presented during the virtual meeting of the Federation of European Neuroscience Societies (FENS) in 2020
List by | Ana Dorrego-Rivas |
ECFG15 – Fungal biology
Preprints presented at 15th European Conference on Fungal Genetics 17-20 February 2020 Rome
List by | Hiral Shah |
ASCB EMBO Annual Meeting 2019
A collection of preprints presented at the 2019 ASCB EMBO Meeting in Washington, DC (December 7-11)
List by | Madhuja Samaddar et al. |
Lung Disease and Regeneration
This preprint list compiles highlights from the field of lung biology.
List by | Rob Hynds |
MitoList
This list of preprints is focused on work expanding our knowledge on mitochondria in any organism, tissue or cell type, from the normal biology to the pathology.
List by | Sandra Franco Iborra |