Induction of cortical Par complex polarity by designed proteins causes cytoskeletal symmetry breaking in unpolarized mammalian cells
Posted on: 17 May 2022
Preprint posted on 16 March 2022
Article now published in Cell at http://dx.doi.org/10.1016/j.cell.2023.08.034
Categories: cell biology
Background to the preprint
Asymmetric division of stem cells allows the formation of cells with different fates: one daughter cell begins to differentiate towards a cell type, whilst the other maintains stem cell identity. The Par complex, discovered in C.elegans and composed of Par3, Par6 and atypical protein kinase C (aPKC), is crucial for the polarisation of cells as it determine the localisation of other polarity proteins and fate determinants (Suzuki & Ohno 2006).
In flies, mechanisms regulating asymmetric division have been extensively studied and are now well known (Knoblich 2008, Knoblich 2010). However, some events during asymmetric division remain elusive, such as symmetry breaking and the cues that trigger the formation of the Par complex. Studies so far are either restricted to flies or limited to single-cell levels (using optogenetics) (Johnston et al 2009, Kono et al 2019, Okumura et al 2018).
In this exciting preprint, the authors use a recently developed in vitro reconstitution assay, which drives the clustering of any protein at the cortex of unpolarised cells. They used this assay to test the ability of wild-type or mutant versions of individual members of the Par complex to i) assemble the entire Par complex and ii) to drive asymmetric cell division in cells that normally divide symmetrically.
Key findings of the preprint
In this study, the authors used a system developed by the lab (Ben-Sasson et al 2021) which allowed them to direct cortical or membranous proteins to spontaneously cluster at the cortex forming caps. Here, the authors chose to study the role of the Par complex and found that inducing the formation of a Par complex cap was sufficient to induce the hallmarks of asymmetric cell division, namely spindle orientation, central spindle asymmetry and the asymmetric segregation of cortical components. Thus, they were able to induce asymmetry in unpolarised cells.
The assembly of the Par complex
First, the authors showed that clustering of any of the Par complex components results in the recruitment of the two other components of the complex at the cortex. Importantly, the lab found that Par6 autoinhibition is the rate-limiting step of the complex assembly. For this, truncated versions of Par6 were expressed in their system, one containing the aPKC binding domain and the other containing the Par3 binding domain. The first construct was able to recruit aPKC faster than the full- length Par6 which shows that Par6 auto-inhibition slows the formation of the Par complex. In addition, the mitochondria-targeted Par6-aPKC binding domain construct was able to recruit cytosolic GFP-Par6-Par3-binding domain construct to the mitochondria and conversely.
These results bring an explanation to a long-standing question in the field, regarding how the Par complex can form despite aPKC inhibitory phosphorylation on Par3 and Par6 inhibition of aPKC kinase activity. Here, the authors showed that the kinase activity of aPKC is mostly dispensable for the assembly of the complex.
Specific roles for each of the Par component
Second, the authors demonstrated that spindle orientation and central spindle asymmetry are controlled via two different pathways. On one hand, Par3 is involved in spindle orientation via mInsc/LGN, complex that, together with NuMA, links the Par complex to microtubules. On the other hand, the kinase activity of aPKC is necessary for symmetry breaking of the central spindle.
Overall, the study concludes that, surprisingly, mouse cells can reproduce several of the hallmarks of asymmetric division that have previously been described in fly: all they need is a Par complex component to cluster.
Method developed using protein design (adapted from Figures 1 and 2). 1. The cells stably express GBP-TM-GBP and the protein of interest fused to GFP. They are then incubated with B(c)GFP and A(d) which trigger clustering. In mitosis, arrays are restricted to one side, and this form an asymmetric cortical cap. 2. Par components, or part of them or mutant versions were fused to GFP and clustered, then the authors looked at the dynamic of the Par complex.
What I liked about the preprint
The team and collaborators designed a very ingenious system that allows symmetry breaking in potentially any cultured cell line! This system could be adapted to other biological questions as potentially any protein at the membrane or the cortex could be used in this system given that protein clustering at the membrane or cortex is not restricted to Par complex.
They now have a tool to study various mechanisms of asymmetric cell division, such as symmetry breaking, the formation and maintenance of other polarity caps, the establishment of central spindle asymmetry but also the cell size asymmetry and the role of cortical tension in division.
The finding that every cell is technically able to divide asymmetrically is very important as this can, in the long term, help us find out how to manipulate cells to divide asymmetrically and form specific cell types.
Future directions
Given the adaptability of the system, there are many directions in which future research could go. There is still more to discover about the Par complex: what is/are the symmetry-breaking event(s)? What triggers the clustering of one Par component leading to the formation of the complex in the first place? Or on the contrary what prevents the formation of the Par caps in symmetrically dividing cells?
Questions for the authors
- What are the mechanisms restricting caps formation to mitosis? It is suggested that caps are formed by increased tension at the cortex or that the caps have a high affinity for zones with high cortical tension. If so, how is Par complex established and/or maintained in interphase in epithelial cells for example?
- How do the authors explain that the different cluster at the cortex assembles into a single large cap in mitosis and not multiple caps spread all over the cortex? Is the location of the cap random?
- Would it be possible to adapt the system to form two caps?
- Would it be possible to change the size of the cap?
References
Ben-Sasson AJ, Watson JL, Sheffler W, Johnson MC, Bittleston A, et al. 2021. Design of biologically active binary protein 2D materials. Nature 589: 468-73
Johnston CA, Hirono K, Prehoda KE, Doe CQ. 2009. Identification of an Aurora-A/PinsLINKER/Dlg spindle orientation pathway using induced cell polarity in S2 cells. Cell 138: 1150-63
Knoblich JA. 2008. Mechanisms of asymmetric stem cell division. Cell 132: 583-97
Knoblich JA. 2010. Asymmetric cell division: recent developments and their implications for tumour biology. Nat Rev Mol Cell Biol 11: 849-60
Kono K, Yoshiura S, Fujita I, Okada Y, Shitamukai A, et al. 2019. Reconstruction of Par-dependent polarity in apolar cells reveals a dynamic process of cortical polarization. Elife 8
Okumura M, Natsume T, Kanemaki MT, Kiyomitsu T. 2018. Dynein-Dynactin-NuMA clusters generate cortical spindle-pulling forces as a multi-arm ensemble. Elife 7
Suzuki A, Ohno S. 2006. The PAR-aPKC system: lessons in polarity. J Cell Sci 119: 979-87
doi: https://doi.org/10.1242/prelights.32029
Read preprintSign up to customise the site to your preferences and to receive alerts
Register hereAlso in the cell biology category:
Motor Clustering Enhances Kinesin-driven Vesicle Transport
Sharvari Pitke
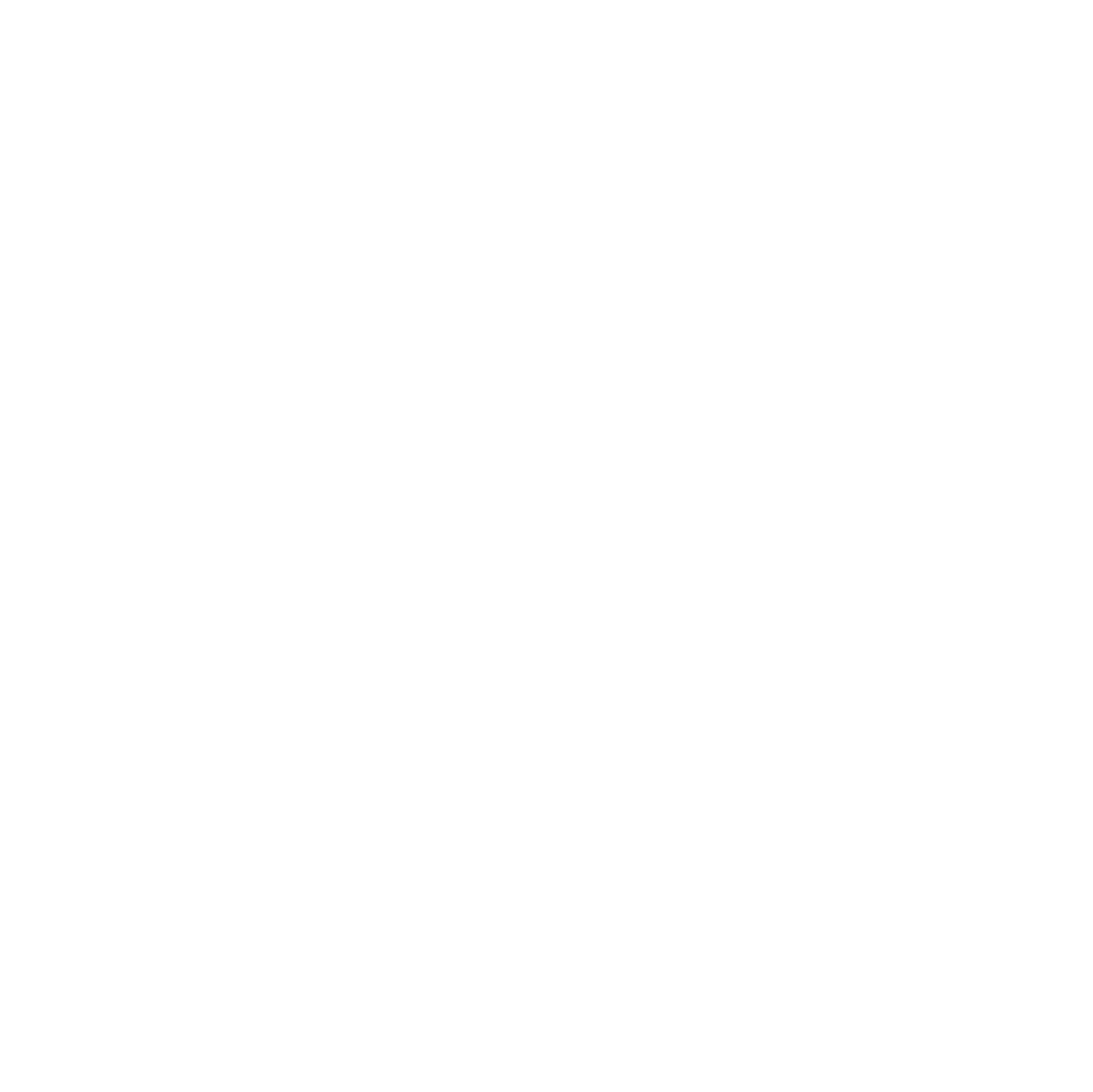
Cellular signalling protrusions enable dynamic distant contacts in spinal cord neurogenesis
Ankita Walvekar
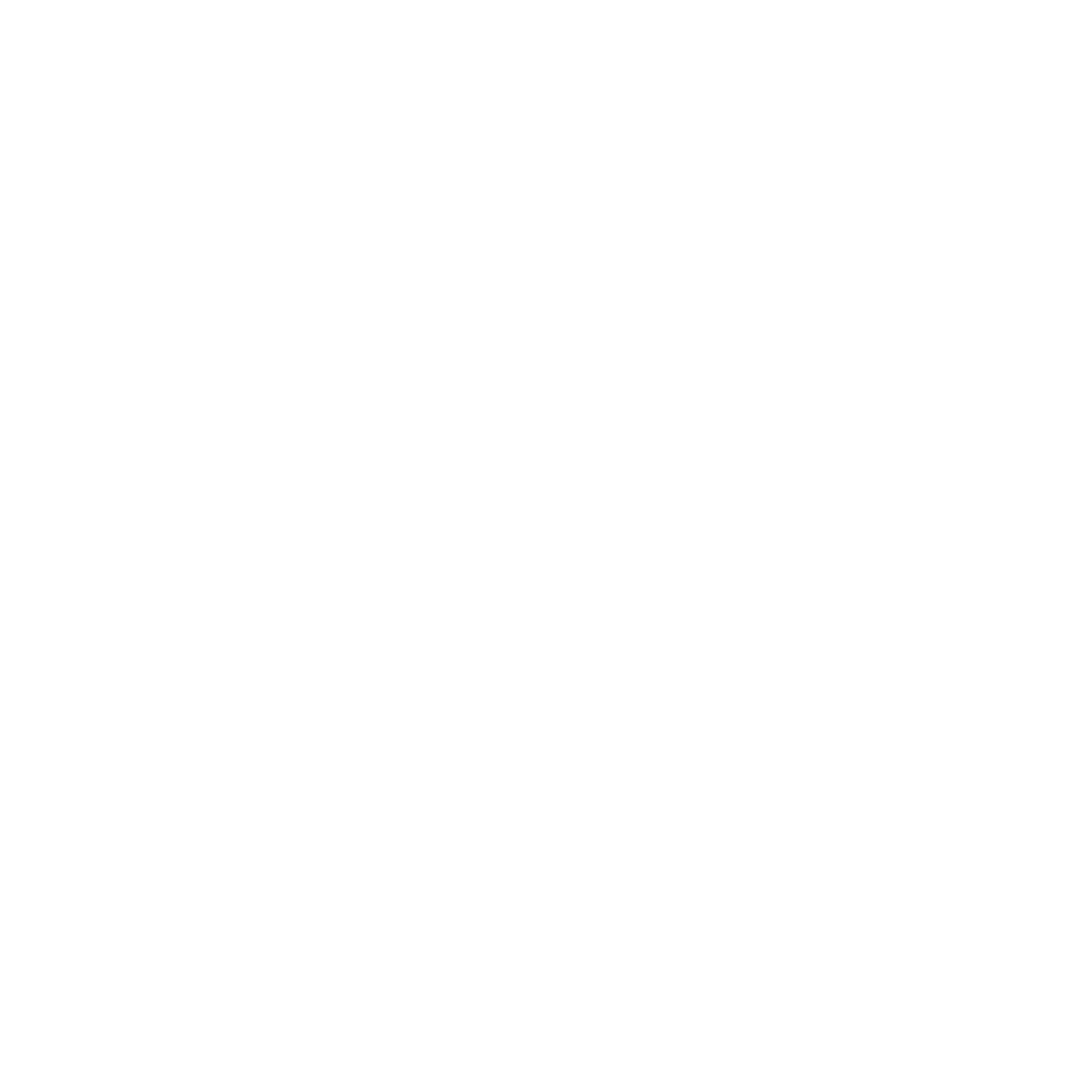
Green synthesized silver nanoparticles from Moringa: Potential for preventative treatment of SARS-CoV-2 contaminated water
Safieh Shah, Benjamin Dominik Maier

preListscell biology category:
in theBSCB-Biochemical Society 2024 Cell Migration meeting
This preList features preprints that were discussed and presented during the BSCB-Biochemical Society 2024 Cell Migration meeting in Birmingham, UK in April 2024. Kindly put together by Sara Morais da Silva, Reviews Editor at Journal of Cell Science.
List by | Reinier Prosee |
‘In preprints’ from Development 2022-2023
A list of the preprints featured in Development's 'In preprints' articles between 2022-2023
List by | Alex Eve, Katherine Brown |
preLights peer support – preprints of interest
This is a preprint repository to organise the preprints and preLights covered through the 'preLights peer support' initiative.
List by | preLights peer support |
The Society for Developmental Biology 82nd Annual Meeting
This preList is made up of the preprints discussed during the Society for Developmental Biology 82nd Annual Meeting that took place in Chicago in July 2023.
List by | Joyce Yu, Katherine Brown |
CSHL 87th Symposium: Stem Cells
Preprints mentioned by speakers at the #CSHLsymp23
List by | Alex Eve |
Journal of Cell Science meeting ‘Imaging Cell Dynamics’
This preList highlights the preprints discussed at the JCS meeting 'Imaging Cell Dynamics'. The meeting was held from 14 - 17 May 2023 in Lisbon, Portugal and was organised by Erika Holzbaur, Jennifer Lippincott-Schwartz, Rob Parton and Michael Way.
List by | Helen Zenner |
9th International Symposium on the Biology of Vertebrate Sex Determination
This preList contains preprints discussed during the 9th International Symposium on the Biology of Vertebrate Sex Determination. This conference was held in Kona, Hawaii from April 17th to 21st 2023.
List by | Martin Estermann |
Alumni picks – preLights 5th Birthday
This preList contains preprints that were picked and highlighted by preLights Alumni - an initiative that was set up to mark preLights 5th birthday. More entries will follow throughout February and March 2023.
List by | Sergio Menchero et al. |
CellBio 2022 – An ASCB/EMBO Meeting
This preLists features preprints that were discussed and presented during the CellBio 2022 meeting in Washington, DC in December 2022.
List by | Nadja Hümpfer et al. |
Fibroblasts
The advances in fibroblast biology preList explores the recent discoveries and preprints of the fibroblast world. Get ready to immerse yourself with this list created for fibroblasts aficionados and lovers, and beyond. Here, my goal is to include preprints of fibroblast biology, heterogeneity, fate, extracellular matrix, behavior, topography, single-cell atlases, spatial transcriptomics, and their matrix!
List by | Osvaldo Contreras |
EMBL Synthetic Morphogenesis: From Gene Circuits to Tissue Architecture (2021)
A list of preprints mentioned at the #EESmorphoG virtual meeting in 2021.
List by | Alex Eve |
FENS 2020
A collection of preprints presented during the virtual meeting of the Federation of European Neuroscience Societies (FENS) in 2020
List by | Ana Dorrego-Rivas |
Planar Cell Polarity – PCP
This preList contains preprints about the latest findings on Planar Cell Polarity (PCP) in various model organisms at the molecular, cellular and tissue levels.
List by | Ana Dorrego-Rivas |
BioMalPar XVI: Biology and Pathology of the Malaria Parasite
[under construction] Preprints presented at the (fully virtual) EMBL BioMalPar XVI, 17-18 May 2020 #emblmalaria
List by | Dey Lab, Samantha Seah |
1
Cell Polarity
Recent research from the field of cell polarity is summarized in this list of preprints. It comprises of studies focusing on various forms of cell polarity ranging from epithelial polarity, planar cell polarity to front-to-rear polarity.
List by | Yamini Ravichandran |
TAGC 2020
Preprints recently presented at the virtual Allied Genetics Conference, April 22-26, 2020. #TAGC20
List by | Maiko Kitaoka et al. |
3D Gastruloids
A curated list of preprints related to Gastruloids (in vitro models of early development obtained by 3D aggregation of embryonic cells). Updated until July 2021.
List by | Paul Gerald L. Sanchez and Stefano Vianello |
ECFG15 – Fungal biology
Preprints presented at 15th European Conference on Fungal Genetics 17-20 February 2020 Rome
List by | Hiral Shah |
ASCB EMBO Annual Meeting 2019
A collection of preprints presented at the 2019 ASCB EMBO Meeting in Washington, DC (December 7-11)
List by | Madhuja Samaddar et al. |
EMBL Seeing is Believing – Imaging the Molecular Processes of Life
Preprints discussed at the 2019 edition of Seeing is Believing, at EMBL Heidelberg from the 9th-12th October 2019
List by | Dey Lab |
Autophagy
Preprints on autophagy and lysosomal degradation and its role in neurodegeneration and disease. Includes molecular mechanisms, upstream signalling and regulation as well as studies on pharmaceutical interventions to upregulate the process.
List by | Sandra Malmgren Hill |
Lung Disease and Regeneration
This preprint list compiles highlights from the field of lung biology.
List by | Rob Hynds |
Cellular metabolism
A curated list of preprints related to cellular metabolism at Biorxiv by Pablo Ranea Robles from the Prelights community. Special interest on lipid metabolism, peroxisomes and mitochondria.
List by | Pablo Ranea Robles |
BSCB/BSDB Annual Meeting 2019
Preprints presented at the BSCB/BSDB Annual Meeting 2019
List by | Dey Lab |
MitoList
This list of preprints is focused on work expanding our knowledge on mitochondria in any organism, tissue or cell type, from the normal biology to the pathology.
List by | Sandra Franco Iborra |
Biophysical Society Annual Meeting 2019
Few of the preprints that were discussed in the recent BPS annual meeting at Baltimore, USA
List by | Joseph Jose Thottacherry |
ASCB/EMBO Annual Meeting 2018
This list relates to preprints that were discussed at the recent ASCB conference.
List by | Dey Lab, Amanda Haage |