Motion of single molecular tethers reveals dynamic subdomains at ER-mitochondria contact sites
Posted on: 6 November 2022
Preprint posted on 3 September 2022
Categories: cell biology
Introduction
Points of contact between the endoplasmic reticulum (ER) and mitochondria are busy hotspots of cellular activity. These membrane contacts are the sites of lipid exchange, mitochondrial fission, ROS signalling, and transfer of calcium which facilitates energy production or, in some cases, cell death.
At contact sites, the ER membrane and outer mitochondrial membrane (OMM) do not directly touch, but are held within 30 nm of one another by reciprocal tethers present within each membrane (1). A well-characterised ER-resident tether is VAMP-associated protein B (VAPB), which, via its N-terminal domain, holds hands with a partner tether present in the OMM such as protein tyrosine phosphatase interacting protein 51 (PTPIP51) (2). Aberrant contact sites have been implicated in various disease states, including metabolic and neurodegenerative diseases. For example, dominant mutations in VAPB are associated with amyotrophic lateral sclerosis (ALS). Although the importance of ER-mitochondria contact sites in health and disease is clear, investigations of these delicate structures have been hampered by technical limitations and experimental artifacts.
In this preprint, Obara and colleagues explore the ultrastructure and organisation of contact sites with minimal perturbation using a complementary set of highly spatially- and temporally-resolved microscopy techniques and sophisticated image analysis. The authors first used focused ion beam-scanning electron microscopy (FIB-SEM) (3) to image the structure of the ER and mitochondria in COS7 cells. For FIB-SEM, a sample is first frozen under high pressure to keep native structures intact, and then an electron beam scans the surface of the sample. A charged ion beam erodes this layer, and the following layer can be imaged, and so on. In this way, 3D renderings of organelles could be reconstructed and their architecture examined. Single particle tracking-photoactivatable localisation microscopy (spt-PALM) (4) is a live-cell super-resolution technique which the authors used to resolve the location of the ER-resident tether, VAPB, beyond the diffraction limit of light. They tagged VAPB with a HaloTag labelled with a photoconvertible fluorophore, which slowly cycles from a dark to a fluorescent state to enable single-molecule localisation. Trajectories of single VAPB molecules moving within the ER membrane could then be tracked, revealing information about the prevalence and diffusion rate of the tether in a particular spatial domain over a time period of seconds.
Key findings
Tethers at contact sites are dynamic
Using 3D reconstructions of FIB-SEM data, the authors identified contact sites where the ER membrane and OMM were within 24 nm of one another. These contact sites were adjacent to functionally-relevant regions of mitochondria: at cristae, the sites of ATP production; and areas of mitochondrial constriction where fission occurs. By examining the curvature of the surface of the ER, the authors showed that the ER curves inwards to fit the mitochondrial surface at the central domain of the contact site, suggesting that these are areas of high adhesive forces which pin the mitochondria and ER together (Figure 1).
Figure 1. 3D FIB-SEM reconstructions of the ER and mitochondria in COS7 cells. Areas of the ER in close contact with the mitochondria are highlighted in red. Adapted from Figure 1a from Obara et al.
By tracking VAPB diffusion within the ER membrane using spt-PALM, the authors showed that most VAPB diffuses freely along ER tubules, but they identified ‘hotspots’ where the probability of VAPB being present was higher. These VAPB hotspots were associated (mostly) with mitochondria, reflecting ER-mitochondria contact sites. They found that the tether diffused into and out of contact sites within seconds, but was both more densely-packed and less mobile at the centre of contact sites (Figure 2). This likely accounts for the central regions of strong adhesion seen in the FIB-SEM reconstructions.
Figure 2. spt-PALM trajectories of single VAPB molecules moving throughout the ER (left). Hotspots where the probability of finding VAPB is high reflect ER-mitochondria contact sites; the density of VAPB is highest in the centre of the contact site (right). Adapted from Figure 3b from Obara et al.
Since the formation of VAPB hotspots was dependent on its N-terminal interactions with its mitochondrial binding partner, PTPIP51, the authors overexpressed this protein to examine how contact sites might be regulated. They found that contact sites became larger – but not more numerous – and VAPB hotspots now associated exclusively with mitochondria. This suggested that the availability of the mitochondrial tether is rate-limiting for the formation of ER-mitochondria contact sites. Since the interaction between VAPB and PTPIP51 is low-affinity, the authors proposed that many binding and unbinding events occur as VAPB diffuses throughout the contact site, implying that tethering at contact sites is very dynamic.
Contact sites adapt to change
Since the behaviour of VAPB is thought to be dynamic, how might tethering at ER-mitochondria contact sites respond to physiological or pathological perturbation?
Again using spt-PALM, the authors showed that starving cells of nutrients led to larger contact sites, where VAPB in the central domain has an even lower diffusion rate than usual. This remodelling probably facilitates metabolite transfer between organelles in response to metabolic stress.
A mutation in the mitochondrial-interacting domain of VAPB (VAPBP56S) is associated with the motor neurone disease ALS. When expressed in cells, some VAPBP56S formed immobile aggregates, but the rest diffused freely in the ER membrane or formed contact sites like the wild-type. However, diffusion of VAPBP56S within contact sites was slower than normal, and the tether became trapped in subdomains rather than diffusing throughout the whole contact site. Interestingly, the slowed diffusion rate in these subdomains was not a result of higher tether density. In addition to the ill effects of protein aggregation contributing to the disease, these stable contact sites might increase inter-organellar calcium transfer and account for the hyperactivity of mitochondria and subsequent oxidative stress also seen in some types of ALS.
Summary
The authors show, using volume electron microscopy and super-resolution single particle tracking, that the ER-resident tether, VAPB, diffuses into and out of ER-mitochondria contact sites and likely forms transient interactions with its mitochondrial binding partner. Within contact sites, tethering proteins are most dense and stable at central regions, which is where adhesion forces between organellar membranes are strongest. Additionally, the authors show that changes to contact site architecture are mediated by changes in tether behaviour under physiological stress or in a disease state.
Why I liked this preprint
My favourite thing about this preprint is that the authors demonstrate the phenomenal resolution achievable using carefully-considered approaches to both light and electron microscopy. No doubt the striking 3D renderings of ER tubules in contact with mitochondria is exciting for any cell biologist to see! I also really like the way the paper is organised, with the core story told succinctly in the main text, accompanied by an extended discussion of the methodology and technical considerations.
Questions for the authors
- You mention that some VAPB probability hotspots are not associated with mitochondria (Fig. 1J). Could these represent contact sites between the ER membrane and other organelles, such as lysosomes (5,6)? Did you examine what happens to these non-mitochondrial contact sites under starvation conditions, when ER-mitochondria contact sites are larger? If they are lost (as they are in the case of PTPIP51 overexpression), could this suggest ER-mitochondria contact sites have priority in states of cellular stress?
- What do you think dictates the density and diffusion rate of VAPB? Would you expect it to be a physical property of the contact site, something controlled by its mitochondrial partner, or the action of other proteins within that region? I found it interesting that there were subdomains of very low VAPBP56S diffusion (Fig. 4J, final panel, blue arrow) which were not necessarily more densely-populated. Do you have any idea why this could be?
References
- Prinz, W. A. (2014). Bridging the gap: Membrane contact sites in signaling, metabolism, and organelle dynamics. Journal of Cell Biology 205, 759–769. https://doi.org/10.1083/jcb.201401126
- De Vos, K. J., Mórotz, G. M., Stoica, R., Tudor, E. L., Lau, K.-F., Ackerley, S., Warley, A., Shaw, C. E. and Miller, C. C. J. (2012). VAPB interacts with the mitochondrial protein PTPIP51 to regulate calcium homeostasis. Human Molecular Genetics 21, 1299–1311. https://doi.org/10.1093/hmg/ddr559
- Xu, C. S., Hayworth, K. J., Lu, Z., Grob, P., Hassan, A. M., García-Cerdán, J. G., Niyogi, K. K., Nogales, E., Weinberg, R. J. and Hess, H. F. (2017). Enhanced FIB-SEM systems for large-volume 3D imaging. eLife 6:e25916. https://doi.org/10.7554/eLife.25916
- Manley, S., Gillette, J. M., Patterson, G. H., Shroff, H., Hess, H. F., Betzig, E. and Lippincott-Schwartz, J. (2008). High-density mapping of single-molecule trajectories with photoactivated localization microscopy. Nature Methods 5, 155–157. https://doi.org/10.1038/nmeth.1176
- Lim, C.-Y., Davis, O. B., Shin, H. R., Zhang, J., Berdan, C. A., Jiang, X., Counihan, J. L., Ory, D. S., Nomura, D. K. and Zoncu, R. (2019). ER–lysosome contacts enable cholesterol sensing by mTORC1 and drive aberrant growth signalling in Niemann–Pick type C. Nature Cell Biology 21, 1206–1218. https://doi.org/10.1038/s41556-019-0391-5
- Özkan, N., Koppers, M., van Soest, I., van Harten, A., Jurriens, D., Liv, N., Klumperman, J., Kapitein, L. C., Hoogenraad, C. C. and Farías, G. G. (2021). ER – lysosome contacts at a pre-axonal region regulate axonal lysosome availability. Nature Communications 12, https://doi.org/10.1038/s41467-021-24713-5
doi: https://doi.org/10.1242/prelights.33066
Read preprintSign up to customise the site to your preferences and to receive alerts
Register hereAlso in the cell biology category:
Motor Clustering Enhances Kinesin-driven Vesicle Transport
Sharvari Pitke
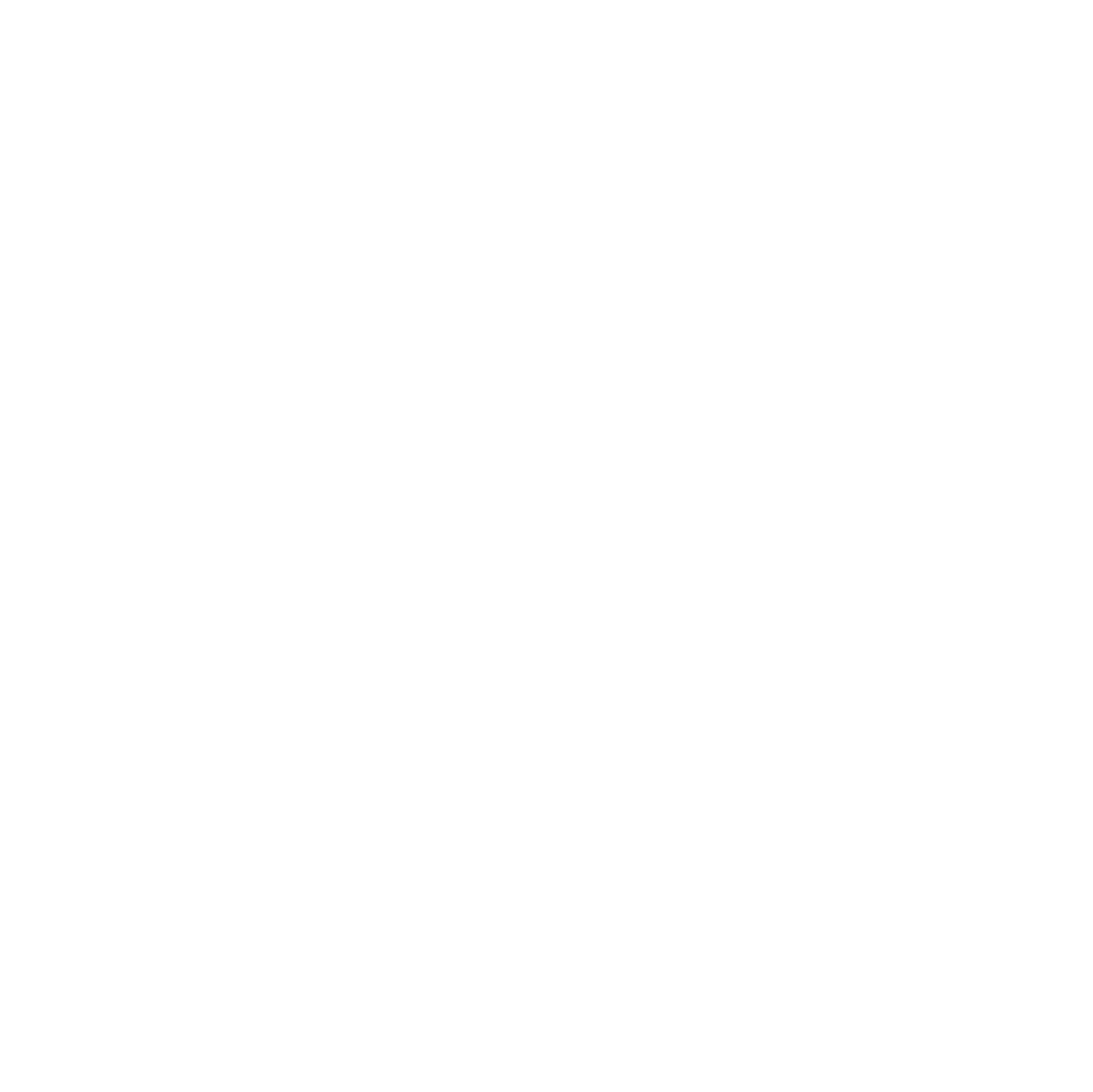
Cellular signalling protrusions enable dynamic distant contacts in spinal cord neurogenesis
Ankita Walvekar
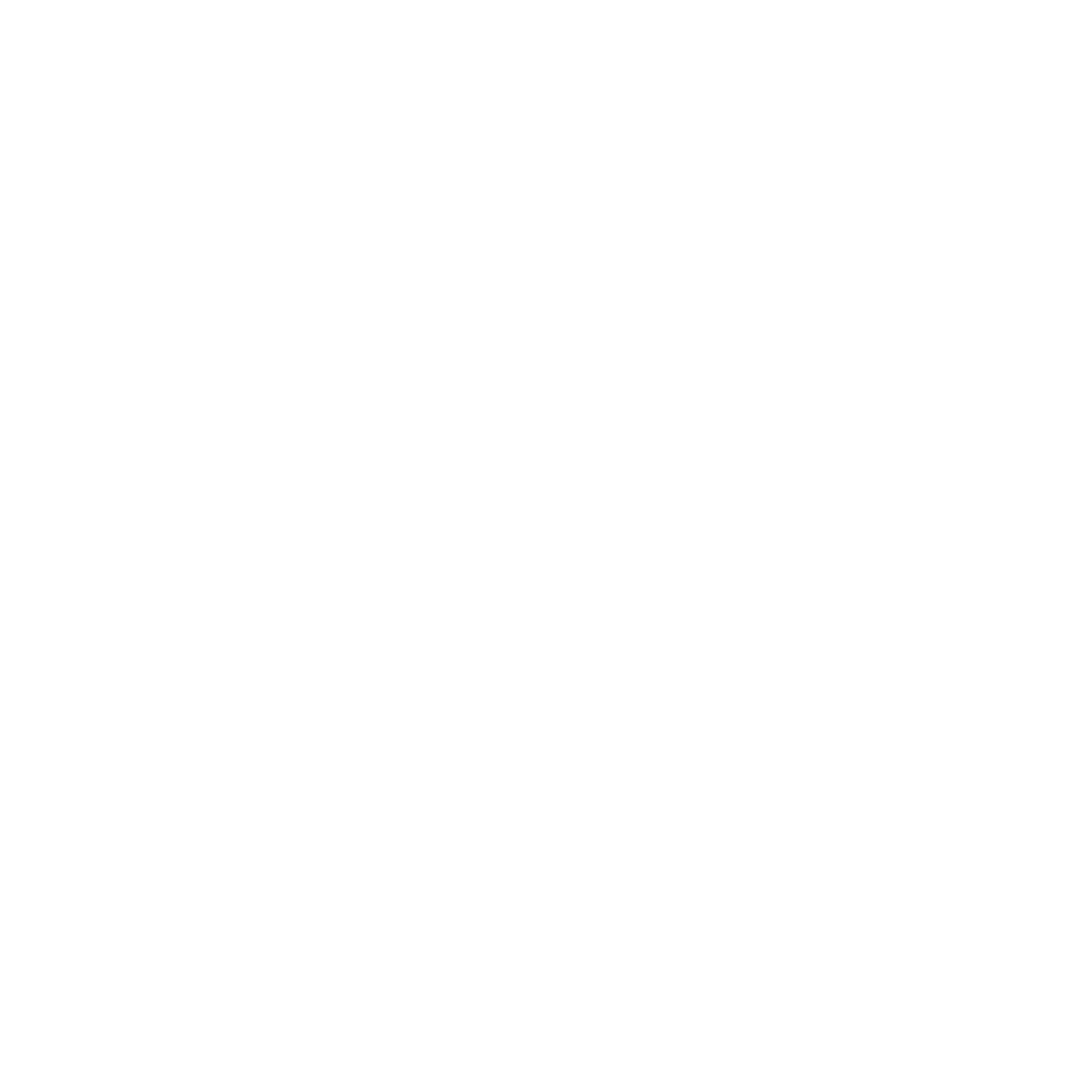
Green synthesized silver nanoparticles from Moringa: Potential for preventative treatment of SARS-CoV-2 contaminated water
Safieh Shah, Benjamin Dominik Maier

preListscell biology category:
in theBSCB-Biochemical Society 2024 Cell Migration meeting
This preList features preprints that were discussed and presented during the BSCB-Biochemical Society 2024 Cell Migration meeting in Birmingham, UK in April 2024. Kindly put together by Sara Morais da Silva, Reviews Editor at Journal of Cell Science.
List by | Reinier Prosee |
‘In preprints’ from Development 2022-2023
A list of the preprints featured in Development's 'In preprints' articles between 2022-2023
List by | Alex Eve, Katherine Brown |
preLights peer support – preprints of interest
This is a preprint repository to organise the preprints and preLights covered through the 'preLights peer support' initiative.
List by | preLights peer support |
The Society for Developmental Biology 82nd Annual Meeting
This preList is made up of the preprints discussed during the Society for Developmental Biology 82nd Annual Meeting that took place in Chicago in July 2023.
List by | Joyce Yu, Katherine Brown |
CSHL 87th Symposium: Stem Cells
Preprints mentioned by speakers at the #CSHLsymp23
List by | Alex Eve |
Journal of Cell Science meeting ‘Imaging Cell Dynamics’
This preList highlights the preprints discussed at the JCS meeting 'Imaging Cell Dynamics'. The meeting was held from 14 - 17 May 2023 in Lisbon, Portugal and was organised by Erika Holzbaur, Jennifer Lippincott-Schwartz, Rob Parton and Michael Way.
List by | Helen Zenner |
9th International Symposium on the Biology of Vertebrate Sex Determination
This preList contains preprints discussed during the 9th International Symposium on the Biology of Vertebrate Sex Determination. This conference was held in Kona, Hawaii from April 17th to 21st 2023.
List by | Martin Estermann |
Alumni picks – preLights 5th Birthday
This preList contains preprints that were picked and highlighted by preLights Alumni - an initiative that was set up to mark preLights 5th birthday. More entries will follow throughout February and March 2023.
List by | Sergio Menchero et al. |
CellBio 2022 – An ASCB/EMBO Meeting
This preLists features preprints that were discussed and presented during the CellBio 2022 meeting in Washington, DC in December 2022.
List by | Nadja Hümpfer et al. |
Fibroblasts
The advances in fibroblast biology preList explores the recent discoveries and preprints of the fibroblast world. Get ready to immerse yourself with this list created for fibroblasts aficionados and lovers, and beyond. Here, my goal is to include preprints of fibroblast biology, heterogeneity, fate, extracellular matrix, behavior, topography, single-cell atlases, spatial transcriptomics, and their matrix!
List by | Osvaldo Contreras |
EMBL Synthetic Morphogenesis: From Gene Circuits to Tissue Architecture (2021)
A list of preprints mentioned at the #EESmorphoG virtual meeting in 2021.
List by | Alex Eve |
FENS 2020
A collection of preprints presented during the virtual meeting of the Federation of European Neuroscience Societies (FENS) in 2020
List by | Ana Dorrego-Rivas |
Planar Cell Polarity – PCP
This preList contains preprints about the latest findings on Planar Cell Polarity (PCP) in various model organisms at the molecular, cellular and tissue levels.
List by | Ana Dorrego-Rivas |
BioMalPar XVI: Biology and Pathology of the Malaria Parasite
[under construction] Preprints presented at the (fully virtual) EMBL BioMalPar XVI, 17-18 May 2020 #emblmalaria
List by | Dey Lab, Samantha Seah |
1
Cell Polarity
Recent research from the field of cell polarity is summarized in this list of preprints. It comprises of studies focusing on various forms of cell polarity ranging from epithelial polarity, planar cell polarity to front-to-rear polarity.
List by | Yamini Ravichandran |
TAGC 2020
Preprints recently presented at the virtual Allied Genetics Conference, April 22-26, 2020. #TAGC20
List by | Maiko Kitaoka et al. |
3D Gastruloids
A curated list of preprints related to Gastruloids (in vitro models of early development obtained by 3D aggregation of embryonic cells). Updated until July 2021.
List by | Paul Gerald L. Sanchez and Stefano Vianello |
ECFG15 – Fungal biology
Preprints presented at 15th European Conference on Fungal Genetics 17-20 February 2020 Rome
List by | Hiral Shah |
ASCB EMBO Annual Meeting 2019
A collection of preprints presented at the 2019 ASCB EMBO Meeting in Washington, DC (December 7-11)
List by | Madhuja Samaddar et al. |
EMBL Seeing is Believing – Imaging the Molecular Processes of Life
Preprints discussed at the 2019 edition of Seeing is Believing, at EMBL Heidelberg from the 9th-12th October 2019
List by | Dey Lab |
Autophagy
Preprints on autophagy and lysosomal degradation and its role in neurodegeneration and disease. Includes molecular mechanisms, upstream signalling and regulation as well as studies on pharmaceutical interventions to upregulate the process.
List by | Sandra Malmgren Hill |
Lung Disease and Regeneration
This preprint list compiles highlights from the field of lung biology.
List by | Rob Hynds |
Cellular metabolism
A curated list of preprints related to cellular metabolism at Biorxiv by Pablo Ranea Robles from the Prelights community. Special interest on lipid metabolism, peroxisomes and mitochondria.
List by | Pablo Ranea Robles |
BSCB/BSDB Annual Meeting 2019
Preprints presented at the BSCB/BSDB Annual Meeting 2019
List by | Dey Lab |
MitoList
This list of preprints is focused on work expanding our knowledge on mitochondria in any organism, tissue or cell type, from the normal biology to the pathology.
List by | Sandra Franco Iborra |
Biophysical Society Annual Meeting 2019
Few of the preprints that were discussed in the recent BPS annual meeting at Baltimore, USA
List by | Joseph Jose Thottacherry |
ASCB/EMBO Annual Meeting 2018
This list relates to preprints that were discussed at the recent ASCB conference.
List by | Dey Lab, Amanda Haage |