Human cardiac fibrosis-on-a-chip model recapitulates disease hallmarks and can serve as a platform for drug screening
Posted on: 24 June 2019
Preprint posted on 13 May 2019
Article now published in Biomaterials at http://dx.doi.org/10.1016/j.biomaterials.2019.119741
Molecular and functional features of cardiac fibrosis pathogenesis recapitulated in vitro: a novel microwell device suited for drug testing.
Selected by Hiroyoshi Y. TanakaCategories: bioengineering, cell biology, physiology
Background:
Cardiac fibrosis is associated with most forms of cardiac disease. Normally, cardiac fibroblasts contribute to the homeostasis of the extracellular matrix. In cardiac fibrosis, however, cardiac fibroblasts are activated in response to injury and become myofibroblasts. Myofibroblasts deposit excessive amounts of extracellular matrix components and eventually replace cardiomyocytes—the cells responsible for the beating of the heart. This negatively affects cardiac function and is related to adverse outcome. However, our understanding of the underlying pathogenetic mechanisms remains limited. This is in part due to a lack of clinically relevant in vitro models. Therefore, the authors set out to create a 3D in vitro model of human cardiac fibrosis in this preprint.
Key findings:
The authors co-cultured human induced pluripotent stem cell (iPSC)-derived cardiomyocytes with cardiac fibroblasts in a microwell device. At both ends of the rectangular microwell are flexible rods placed parallel to each other. The contraction of the cultured cardiomyocytes causes a deflection of the rods. Real-time monitoring of rod deflection allows the measurement of contractile force. To generate the human cardiac fibrosis-on-a-chip (hCF-on-a-chip) model, the authors used the profibrotic agent Transforming Growth Factor (TGF) β1. Cardiac fibroblasts were first activated with TGFβ1 before co-culture. TGFβ1 was further added to the culture medium during 3 weeks of co-culture within the microwell device to promote a fibrotic phenotype.
The authors performed a comprehensive analysis of both molecular and functional changes that occur in the hCF-on-a-chip as compared to its healthy counterpart (without TGFβ1 treatment). Molecularly, the authors observed the increased presence of α-smooth muscle actin (αSMA) positive myofibroblasts, increased collagen I density, increased Brain Natriuretic Peptide (BNP) secretion, as well as a transcriptomic (mRNA and miRNA) profile consistent with fibrosis in the hCF-on-a-chip. These changes were paralleled by increased tissue stiffness, which is also a hallmark of fibrotic pathology. All of these changes were accompanied by alterations in cardiomyocyte function such as increased passive tension, decreased contractile force, and an elevated excitation threshold.
Furthermore, the suitability of hCF-on-a-chip in studying drug efficacy was tested. The hCF-on-a-chip responded to clinical drugs (losartan and carvedilol) in a manner consistent with what is seen in human patients. Notably, the hCF-on-a-chip recapitulated therapeutic responses seen in human patients better than conventional 2D culture. This is in line with a growing body of evidence suggesting that higher-order intercellular interaction is critical for cellular function, both in health and in disease. The authors finally tested the efficacy of pirfenidone, an anti-fibrotic used for the treatment of idiopathic pulmonary fibrosis. Several measures of fibrosis were ameliorated by pirfenidone treatment.
What I like about this preprint & why I think the work is important:
The strength of this model is its adaptability to various readouts such as microscopic analysis, transcriptomics, mechanical measurements, and assessment of contractile function, just to name a few that are performed in the manuscript. The ability to perform multi-modal measurements (at a spatiotemporal resolution that would otherwise be difficult in vivo) will allow integration of various findings reported in isolation to date. It will moreover likely fuel a more systems approach in unraveling the mechanisms driving fibrosis and functional deterioration of cardiomyocytes. The hCF-on-a-chip, combined with various innovative analytical strategies, will greatly benefit our understanding of cardiac fibrosis.
Future directions & questions for the authors:
Q1. The present hCF-on-a-chip was generated by co-culturing iPSC-derived cardiomyocytes with generic cardiac fibroblasts. With patient-specific testing in mind, could the model perhaps be fully iPSC-derived?
Q2. The authors demonstrate a proof-of-concept for the use of these hCF-on-a-chips for drug testing. How is the scalability of these chips for drug screening?
Q3. Another important factor in the induction and/or progression of cardiac fibrosis is abnormal hemodynamics. Could abnormal flow perhaps be modeled in the hCF-on-a-chip?
Q4. TGFβ1 was used to induce a fibrotic phenotype in the present model. Are there any other ways to induce a fibrotic reaction in the hCF-on-a-chip that does not rely on the administration of TGFβ?
Q5. The authors mainly reported results after 2 or 3 weeks of TGFβ1 treatment. Would it be possible to see the temporal dynamics of disease progression (for example, how the extracellular matrix becomes remodeled over time and how it correlates with the decline of cardiomyocyte function)? On a related note, for how long can these chips be sustained in culture?
Q6. The authors observed spatial heterogeneity within the hCF-on-a-chip, specifically the heterogeneous distribution of tissue stiffness. What are the mechanisms driving such heterogeneity (especially given that TGFβ1 was uniformly administered)?
doi: https://doi.org/10.1242/prelights.11372
Read preprintSign up to customise the site to your preferences and to receive alerts
Register hereAlso in the bioengineering category:
Scalable and efficient generation of mouse primordial germ cell-like cells
Carly Guiltinan

Generalized Biomolecular Modeling and Design with RoseTTAFold All-Atom
Saanjbati Adhikari

Multi-pass, single-molecule nanopore reading of long protein strands with single-amino acid sensitivity
Benjamin Dominik Maier, Samantha Seah

Also in the cell biology category:
Cell cycle-dependent mRNA localization in P-bodies
Mohammed JALLOH

Control of Inflammatory Response by Tissue Microenvironment
Roberto Amadio
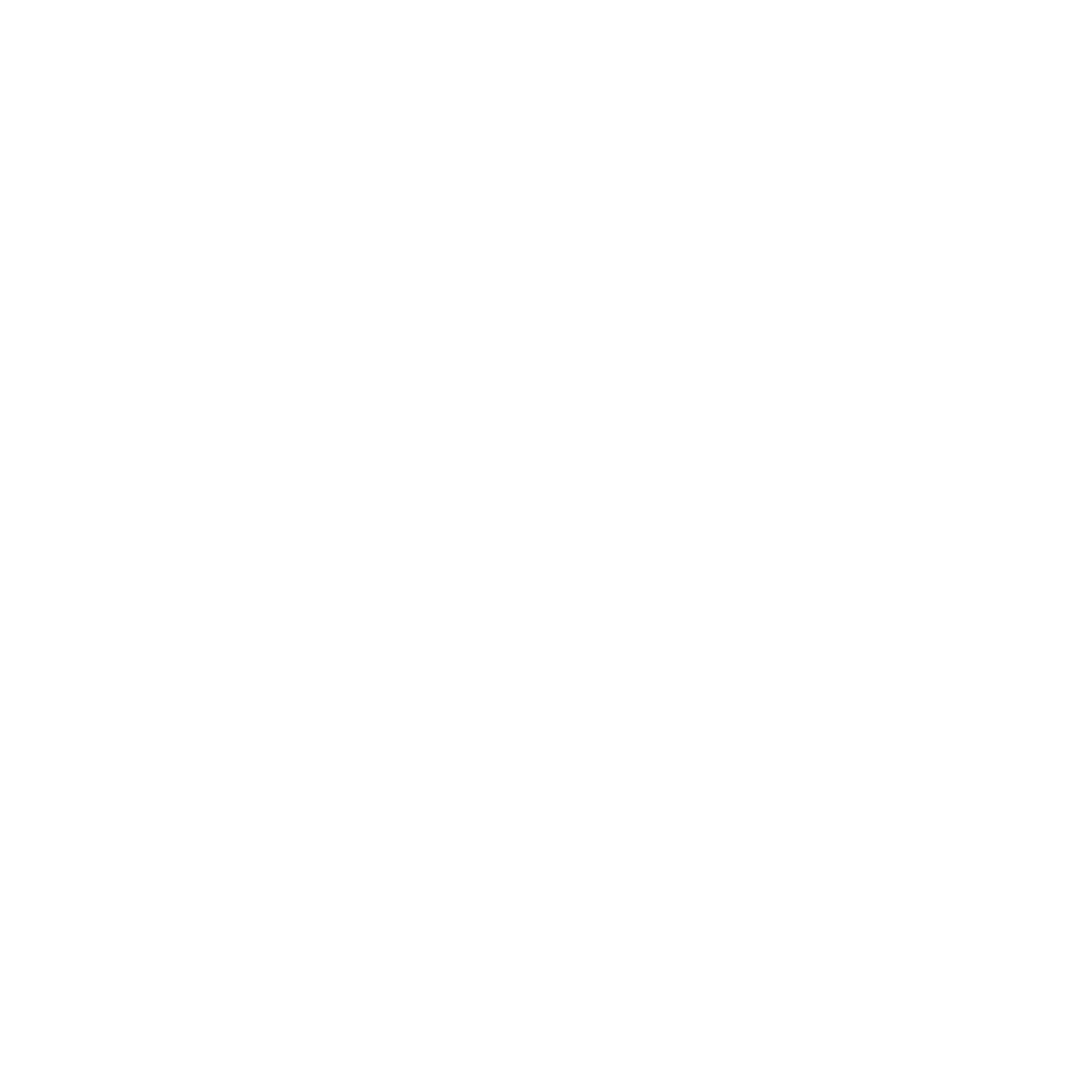
Notch3 is a genetic modifier of NODAL signalling for patterning asymmetry during mouse heart looping
Bhaval Parmar
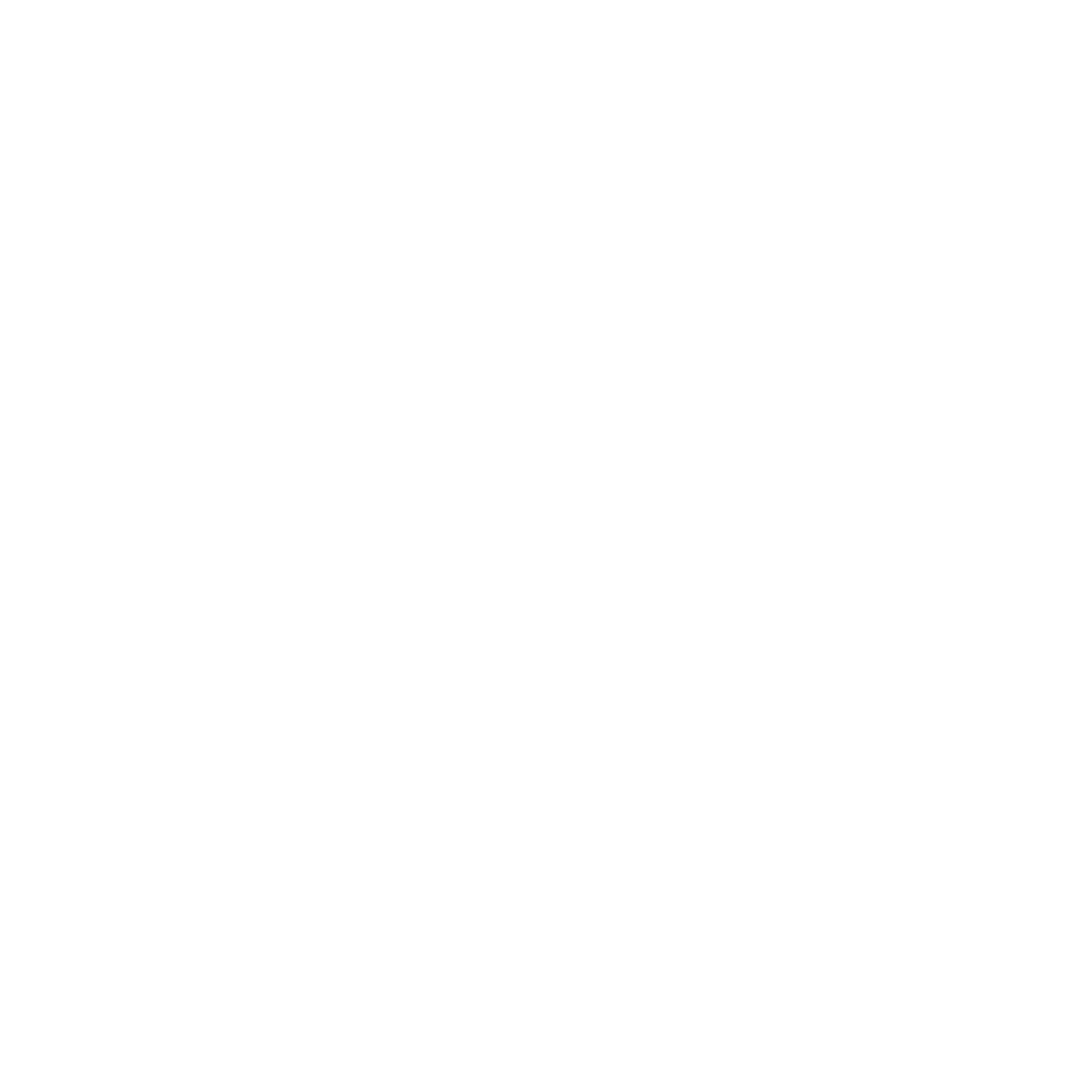
Also in the physiology category:
Gestational exposure to high heat-humidity conditions impairs mouse embryonic development
Girish Kale, preLights peer support
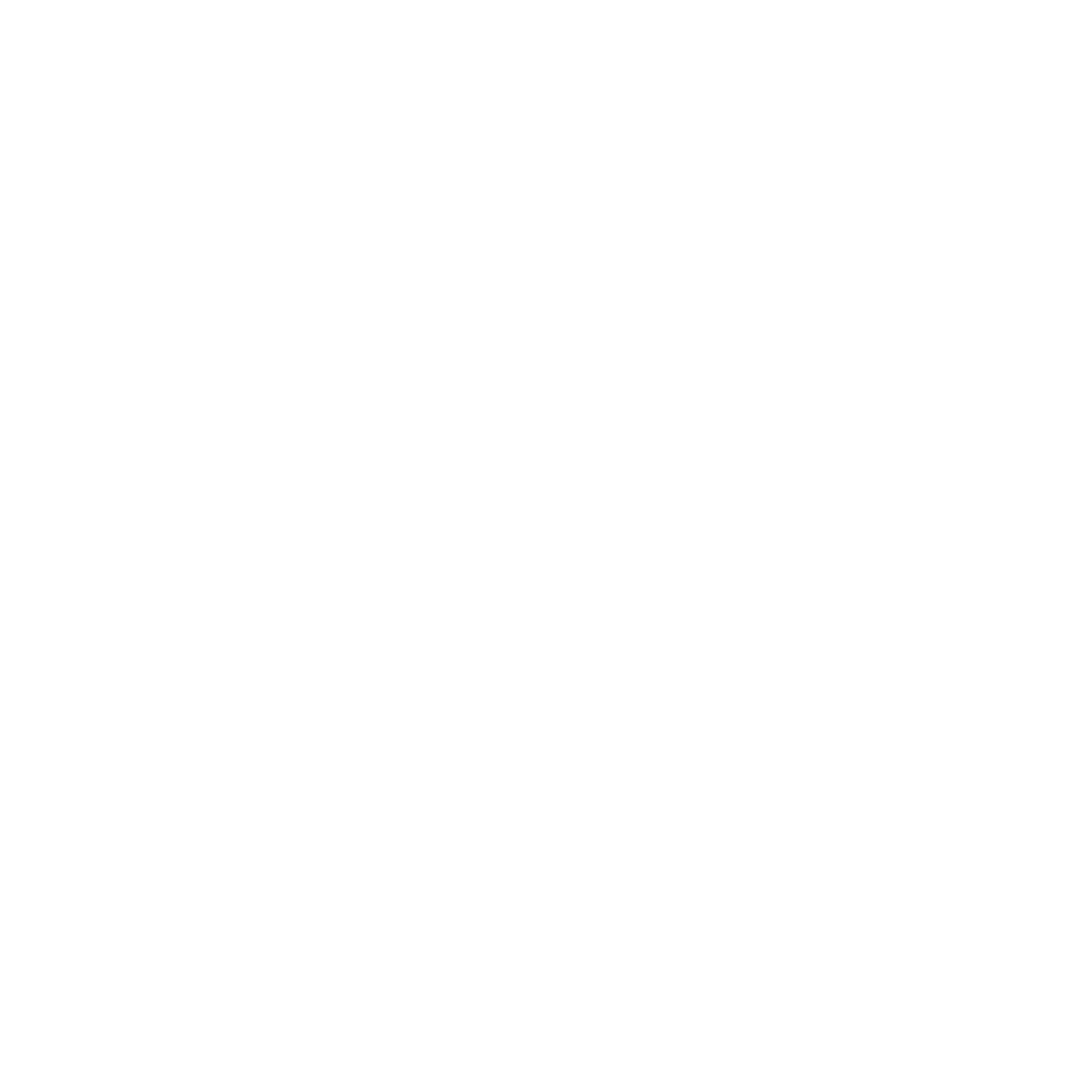
Modular control of time and space during vertebrate axis segmentation
AND
Natural genetic variation quantitatively regulates heart rate and dimension
Girish Kale, Jennifer Ann Black
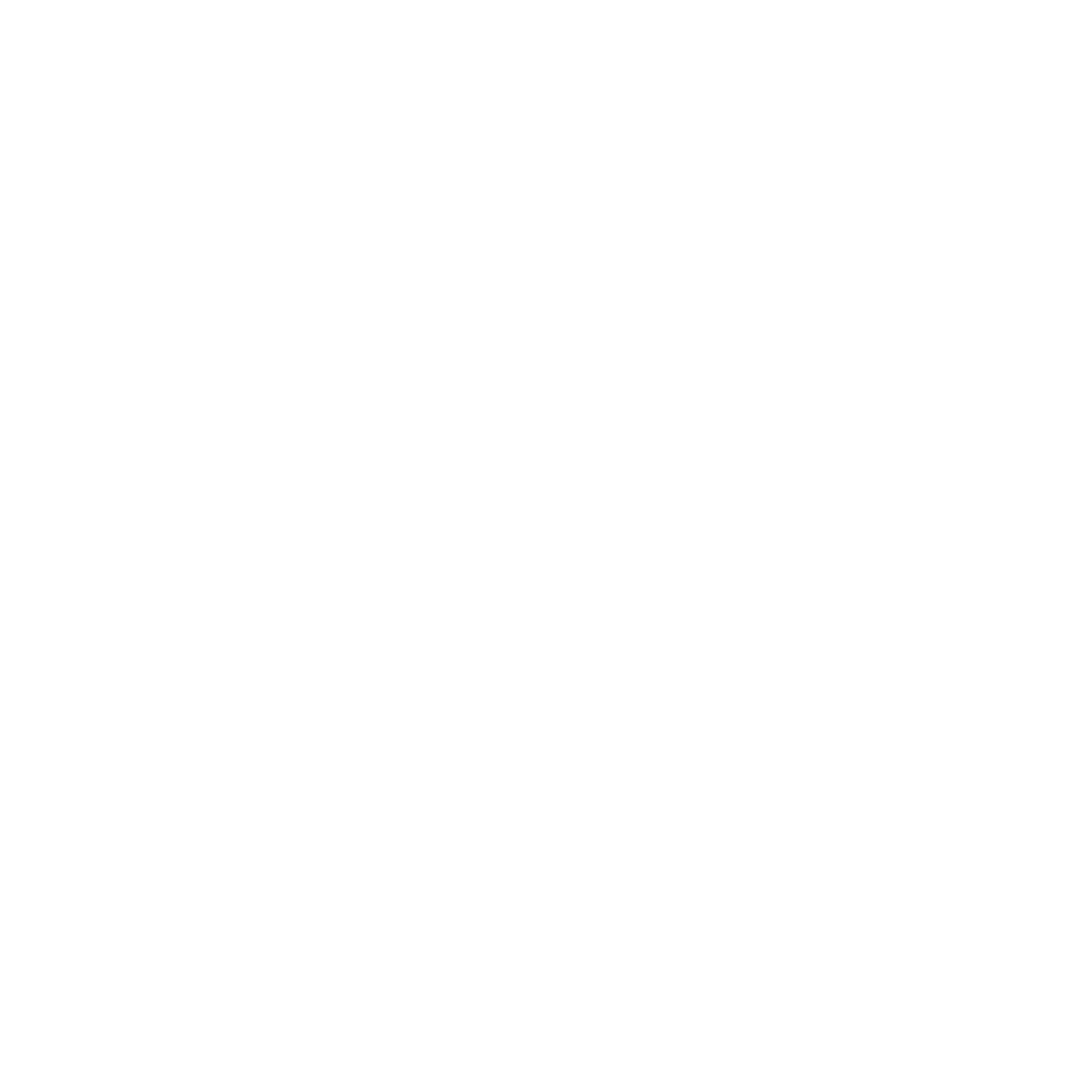
Blue appendages and temperature acclimation increase survival during acute heat stress in the upside-down jellyfish, Cassiopea xamachana
Maitri Manjunath

preListsbioengineering category:
in theCSHL 87th Symposium: Stem Cells
Preprints mentioned by speakers at the #CSHLsymp23
List by | Alex Eve |
EMBL Synthetic Morphogenesis: From Gene Circuits to Tissue Architecture (2021)
A list of preprints mentioned at the #EESmorphoG virtual meeting in 2021.
List by | Alex Eve |
3D Gastruloids
A curated list of preprints related to Gastruloids (in vitro models of early development obtained by 3D aggregation of embryonic cells). Updated until July 2021.
List by | Paul Gerald L. Sanchez and Stefano Vianello |
ASCB EMBO Annual Meeting 2019
A collection of preprints presented at the 2019 ASCB EMBO Meeting in Washington, DC (December 7-11)
List by | Madhuja Samaddar et al. |
EMBL Seeing is Believing – Imaging the Molecular Processes of Life
Preprints discussed at the 2019 edition of Seeing is Believing, at EMBL Heidelberg from the 9th-12th October 2019
List by | Dey Lab |
Lung Disease and Regeneration
This preprint list compiles highlights from the field of lung biology.
List by | Rob Hynds |
Advances in microscopy
This preList highlights exciting unpublished preprint articles describing advances in microscopy with a focus on light-sheet microscopy.
List by | Stephan Daetwyler |
Also in the cell biology category:
BSCB-Biochemical Society 2024 Cell Migration meeting
This preList features preprints that were discussed and presented during the BSCB-Biochemical Society 2024 Cell Migration meeting in Birmingham, UK in April 2024. Kindly put together by Sara Morais da Silva, Reviews Editor at Journal of Cell Science.
List by | Reinier Prosee |
‘In preprints’ from Development 2022-2023
A list of the preprints featured in Development's 'In preprints' articles between 2022-2023
List by | Alex Eve, Katherine Brown |
preLights peer support – preprints of interest
This is a preprint repository to organise the preprints and preLights covered through the 'preLights peer support' initiative.
List by | preLights peer support |
The Society for Developmental Biology 82nd Annual Meeting
This preList is made up of the preprints discussed during the Society for Developmental Biology 82nd Annual Meeting that took place in Chicago in July 2023.
List by | Joyce Yu, Katherine Brown |
CSHL 87th Symposium: Stem Cells
Preprints mentioned by speakers at the #CSHLsymp23
List by | Alex Eve |
Journal of Cell Science meeting ‘Imaging Cell Dynamics’
This preList highlights the preprints discussed at the JCS meeting 'Imaging Cell Dynamics'. The meeting was held from 14 - 17 May 2023 in Lisbon, Portugal and was organised by Erika Holzbaur, Jennifer Lippincott-Schwartz, Rob Parton and Michael Way.
List by | Helen Zenner |
9th International Symposium on the Biology of Vertebrate Sex Determination
This preList contains preprints discussed during the 9th International Symposium on the Biology of Vertebrate Sex Determination. This conference was held in Kona, Hawaii from April 17th to 21st 2023.
List by | Martin Estermann |
Alumni picks – preLights 5th Birthday
This preList contains preprints that were picked and highlighted by preLights Alumni - an initiative that was set up to mark preLights 5th birthday. More entries will follow throughout February and March 2023.
List by | Sergio Menchero et al. |
CellBio 2022 – An ASCB/EMBO Meeting
This preLists features preprints that were discussed and presented during the CellBio 2022 meeting in Washington, DC in December 2022.
List by | Nadja Hümpfer et al. |
Fibroblasts
The advances in fibroblast biology preList explores the recent discoveries and preprints of the fibroblast world. Get ready to immerse yourself with this list created for fibroblasts aficionados and lovers, and beyond. Here, my goal is to include preprints of fibroblast biology, heterogeneity, fate, extracellular matrix, behavior, topography, single-cell atlases, spatial transcriptomics, and their matrix!
List by | Osvaldo Contreras |
EMBL Synthetic Morphogenesis: From Gene Circuits to Tissue Architecture (2021)
A list of preprints mentioned at the #EESmorphoG virtual meeting in 2021.
List by | Alex Eve |
FENS 2020
A collection of preprints presented during the virtual meeting of the Federation of European Neuroscience Societies (FENS) in 2020
List by | Ana Dorrego-Rivas |
Planar Cell Polarity – PCP
This preList contains preprints about the latest findings on Planar Cell Polarity (PCP) in various model organisms at the molecular, cellular and tissue levels.
List by | Ana Dorrego-Rivas |
BioMalPar XVI: Biology and Pathology of the Malaria Parasite
[under construction] Preprints presented at the (fully virtual) EMBL BioMalPar XVI, 17-18 May 2020 #emblmalaria
List by | Dey Lab, Samantha Seah |
1
Cell Polarity
Recent research from the field of cell polarity is summarized in this list of preprints. It comprises of studies focusing on various forms of cell polarity ranging from epithelial polarity, planar cell polarity to front-to-rear polarity.
List by | Yamini Ravichandran |
TAGC 2020
Preprints recently presented at the virtual Allied Genetics Conference, April 22-26, 2020. #TAGC20
List by | Maiko Kitaoka et al. |
3D Gastruloids
A curated list of preprints related to Gastruloids (in vitro models of early development obtained by 3D aggregation of embryonic cells). Updated until July 2021.
List by | Paul Gerald L. Sanchez and Stefano Vianello |
ECFG15 – Fungal biology
Preprints presented at 15th European Conference on Fungal Genetics 17-20 February 2020 Rome
List by | Hiral Shah |
ASCB EMBO Annual Meeting 2019
A collection of preprints presented at the 2019 ASCB EMBO Meeting in Washington, DC (December 7-11)
List by | Madhuja Samaddar et al. |
EMBL Seeing is Believing – Imaging the Molecular Processes of Life
Preprints discussed at the 2019 edition of Seeing is Believing, at EMBL Heidelberg from the 9th-12th October 2019
List by | Dey Lab |
Autophagy
Preprints on autophagy and lysosomal degradation and its role in neurodegeneration and disease. Includes molecular mechanisms, upstream signalling and regulation as well as studies on pharmaceutical interventions to upregulate the process.
List by | Sandra Malmgren Hill |
Lung Disease and Regeneration
This preprint list compiles highlights from the field of lung biology.
List by | Rob Hynds |
Cellular metabolism
A curated list of preprints related to cellular metabolism at Biorxiv by Pablo Ranea Robles from the Prelights community. Special interest on lipid metabolism, peroxisomes and mitochondria.
List by | Pablo Ranea Robles |
BSCB/BSDB Annual Meeting 2019
Preprints presented at the BSCB/BSDB Annual Meeting 2019
List by | Dey Lab |
MitoList
This list of preprints is focused on work expanding our knowledge on mitochondria in any organism, tissue or cell type, from the normal biology to the pathology.
List by | Sandra Franco Iborra |
Biophysical Society Annual Meeting 2019
Few of the preprints that were discussed in the recent BPS annual meeting at Baltimore, USA
List by | Joseph Jose Thottacherry |
ASCB/EMBO Annual Meeting 2018
This list relates to preprints that were discussed at the recent ASCB conference.
List by | Dey Lab, Amanda Haage |
Also in the physiology category:
Fibroblasts
The advances in fibroblast biology preList explores the recent discoveries and preprints of the fibroblast world. Get ready to immerse yourself with this list created for fibroblasts aficionados and lovers, and beyond. Here, my goal is to include preprints of fibroblast biology, heterogeneity, fate, extracellular matrix, behavior, topography, single-cell atlases, spatial transcriptomics, and their matrix!
List by | Osvaldo Contreras |
FENS 2020
A collection of preprints presented during the virtual meeting of the Federation of European Neuroscience Societies (FENS) in 2020
List by | Ana Dorrego-Rivas |
TAGC 2020
Preprints recently presented at the virtual Allied Genetics Conference, April 22-26, 2020. #TAGC20
List by | Maiko Kitaoka et al. |
Autophagy
Preprints on autophagy and lysosomal degradation and its role in neurodegeneration and disease. Includes molecular mechanisms, upstream signalling and regulation as well as studies on pharmaceutical interventions to upregulate the process.
List by | Sandra Malmgren Hill |
Cellular metabolism
A curated list of preprints related to cellular metabolism at Biorxiv by Pablo Ranea Robles from the Prelights community. Special interest on lipid metabolism, peroxisomes and mitochondria.
List by | Pablo Ranea Robles |