Microglia integration into human midbrain organoids leads to increased neuronal maturation and functionality
Posted on: 8 March 2022
Preprint posted on 22 January 2022
Article now published in Glia at http://dx.doi.org/10.1002/glia.24167
Ever wondered how we can model the human brain in a dish? Organoids and assembloids mimic the structure and complexity of the human brain!
Selected by Emma WilsonCategories: cell biology, neuroscience
Introduction
The human brain is a highly complex organ in terms of structure molecular and cellular composition, making neurological disorders difficult to model. The recent emergence of 3D cellular cultures called organoids has been revolutionary in mimicking the spatial and functional complexity of the human brain, and a variety of organoid differentiation protocols have been developed to try and model the diverse range of neurological disorders1,2. Interestingly, many labs have been focusing on generating midbrain organoids. Currently, midbrain organoids have been generated in both patient and non-patient cells and have been able to recapitulate cardinal features of Parkinson’s disease (PD)3,4. These midbrain organoids have key hallmarks of PD including loss of dopaminergic neurons and protein aggregation, highlighting these as a potential model.
One of the major disadvantages of the current midbrain organoids differentiation protocols is the lack microglia. Microglia are the largest population of immune cells in the brain. They are tissue-resident macrophages and represent 5-15% of all adult brain cells. Microglia function to establish contacts with neural progenitors to support neurogenesis during development and interact with neurons, astrocytes, and oligodendrocytes to help brain homeostasis and immune defence5. Organoids lacking microglia are not able to model neurological disorders appropriately as neuroinflammation is a major feature of many disorders including Parkinson’s Disease (PD)6.
In this preprint, the authors describe a protocol to generate assembloids; organoids which have incorporated microglia to allow a truer model of PD and other neurological disorders.
Generating Assembloids
The authors of this preprint combined protocols for microglia differentiation and midbrain organoid differentiation. First, they tested midbrain organoid differentiation media, microglia media and a combination of microglia media with essential midbrain organoid differentiation factors (co-culture medium)(Figure 1a). They found that the co-culture medium showed no differences in the viability of the microglia compared to the microglia media alone, therefore suggesting that they can be co-cultured with midbrain organoids without loss of microglia viability or changes in midbrain maturation.
During the co-culture protocol the macroglia precursors first aggregate to form smaller, round colonies that attached to the surface of the organoid. The microglia are then incorporated within the structure. After 20 days of co-culture, the incorporation of microglia into the midbrain organoid was assessed via immunofluorescence of IBA1, a reliable microglia marker. The authors found 6.4% of cells were IBA1 positives, which is within the normal physiological range for this cell type. Neuronal populations within the assembloid were confirmed by pan-neuronal markers MAP2 and TUJ1 and midbrain identity was confirmed via FOX2A, a dopaminergic precursor marker and TH, a dopaminergic neurons marker (Figure 1b). Interestingly, after 70 days of culture the presence of GFAP, an astrocytic marker, was found in the population. This suggests that microglia have integrated into the midbrain organoid and all expected cell types are present. This was further confirmed using single cell RNA (scRNA) sequencing. scRNA sequencing showed a range of cell populations (8 in total) in the assembloid including mature dopaminergic, GABAergic neurones as well as microglia (Figure 1c). However, the authors found only 1% of cells were microglia, which was lower than the expected 6% as shown previously via immunofluorescence. They authors reasoned this was due to the loss of cells in the extraction of RNA for RNA sequencing. The 1% of microglia that were present did have a distinct RNA expression pattern, different from the neuronal or precursor populations and the top 100 microglia markers were present, giving confidence that these were true microglia.
Next, they examined the function of the microglia. Activated microglia at the site of inflammation change their morphology and become phagocytic. They release inflammatory cytokines that amplify the inflammatory response by activating and recruiting other cells to the brain lesion7. The media in which the assembloids were growing contained excreted cytokines and chemokines, suggesting that the microglia were functional. This data suggests that midbrain organoids can reliability incorporate microglia and are functional in assembloids.
Figure 1: a) Schematic diagram showing the process of generating assembloids and testing the various medias. B) immunofluorescent images of assembloids showing nucleoid (blue) microglia marker IBA1 (green) and FOXA2 or TH (red) OR MAP2 and TUJ1 (white). C) single cell RNA sequencing data showing the different cell types present in midbrain organoids and assembloids as shows as a percentage.
What is the effect of microglia on midbrain organoids?
After generating a reliable model for assembloids the authors then assessed what the impact of the microglia were on the neural cells within the assembloids. Firstly, the assembloids were shown to express phagocytic genes, indicating that they are functional. The assembloids also appeared smaller with a significantly reduced amount of dead cells compared to organoids, implying that the microglia are removing dead cells. Next, the authors examined differentially expressed genes in assembloids compared to midbrain organoids. They found 12 differentially expressed pathways that included changes to oxidative stress genes, immunoresponse genes, and neurogenesis and axonal guidance genes. Of particular interest were differentially expressed genes involved with synaptic vesicle exocytosis, synaptic contact or synaptogenesis. General synaptic markers such as synaptotagmin and synaptophysin were down regulated across all the cell types in the assembloids, as were dopaminergic neuronal circuit formation genes ROBO1 and DCC. This suggests that axonal remodelling is influenced by the presence of microglia. To examine this further the authors used patch clamping to assess the functional impact of these alterations in gene and protein expression. They found that neurons in midbrain organoids and assembloids exhibit similar
- Resting membrane potentials and input resistance
- Reliable fired repetitive action potentials
- Amplitude of these currents were not different between both groups.
The voltage threshold for action potential generation, however, was more negative in the group of assembloids neurons, which is common and a strong indicator of increased neuronal excitability as seen in mature neurons. They followed this up using a multi electrode array platform (MEA) which can recode action potential across a population of cells. The MEA data showed a lower inter spike interval, meaning the distance between action potentials is smaller and supporting what was observed in the patch clamp experiments.
In summary, neurons in assembloids develop fully mature electrophysiological properties with a lower threshold for action potential generation than in midbrain organoids.
Why I chose this preprint
As a researcher into neurodegenerative diseases, I found it highly intriguing that we may be able to model Parkinson’s disease and other neurodegenerative disease in a 3D cell culture model. This will allow us researchers to test new drugs and treatments in vitro in a human model and only send those which are most promising to human trial. This would hopefully reduce the number of mice used in research as well increase the amount of drug targets which transfer to patients. This is another great tool in our scientific tool kit. The incorporation of other cell types also opens interesting fields or research about combining different organoids.
Questions
- Why did you use nuclei rather than whole cells for your single cell RNAseq? Was there an experimental issue with making them single cells? scRNA also showed less microglia than expected? Why do you think this was?
- After 70 days in culture you got astrocytes, was this expected and what does that mean?
- Why do you think having microglia reduces the formation of synapses or alters synapse formation?
- You showed that six of the strongly downregulated genes were involved with oxidative stress? Do you have a theory as to why these were downregulated?
References
- Choi, S. H. et al. A three-dimensional human neural cell culture model of Alzheimer’s disease. Nature 515, (2014).
- Lancaster, M. A. et al. Cerebral organoids model human brain development and microcephaly. Nature 501, (2013).
- Smits, L. M. et al. Modeling Parkinson’s disease in midbrain-like organoids. npj Park. Dis. 5, (2019).
- Kim, H. et al. Modeling G2019S-LRRK2 Sporadic Parkinson’s Disease in 3D Midbrain Organoids. Stem Cell Reports 12, (2019).
- Thion, M. S., Ginhoux, F. & Garel, S. Microglia and early brain development: An intimate journey. Science 362, (2018).
- Kouli, A., Camacho, M., Allinson, K. & Williams-Gray, C. H. Neuroinflammation and protein pathology in Parkinson’s disease dementia. Acta Neuropathol. Commun. 8, (2020).
- Kim, Y. S. & Joh, T. H. Microglia, major player in the brain inflammation: Their roles in the pathogenesis of Parkinson’s disease. Experimental and Molecular Medicine 38, (2006).
doi: https://doi.org/10.1242/prelights.31489
Read preprintSign up to customise the site to your preferences and to receive alerts
Register hereAlso in the cell biology category:
Cell cycle-dependent mRNA localization in P-bodies
Mohammed JALLOH

Control of Inflammatory Response by Tissue Microenvironment
Roberto Amadio
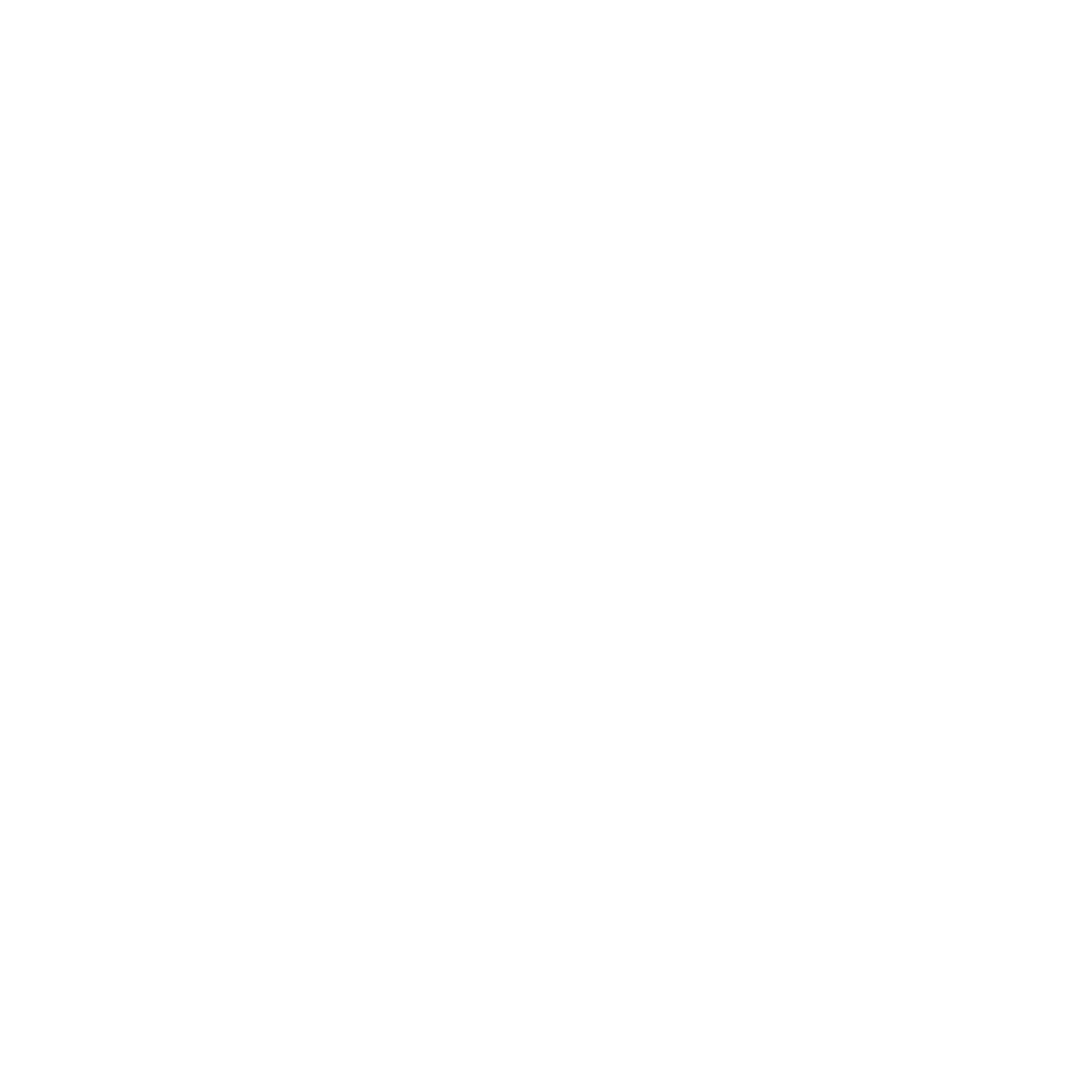
Notch3 is a genetic modifier of NODAL signalling for patterning asymmetry during mouse heart looping
Bhaval Parmar
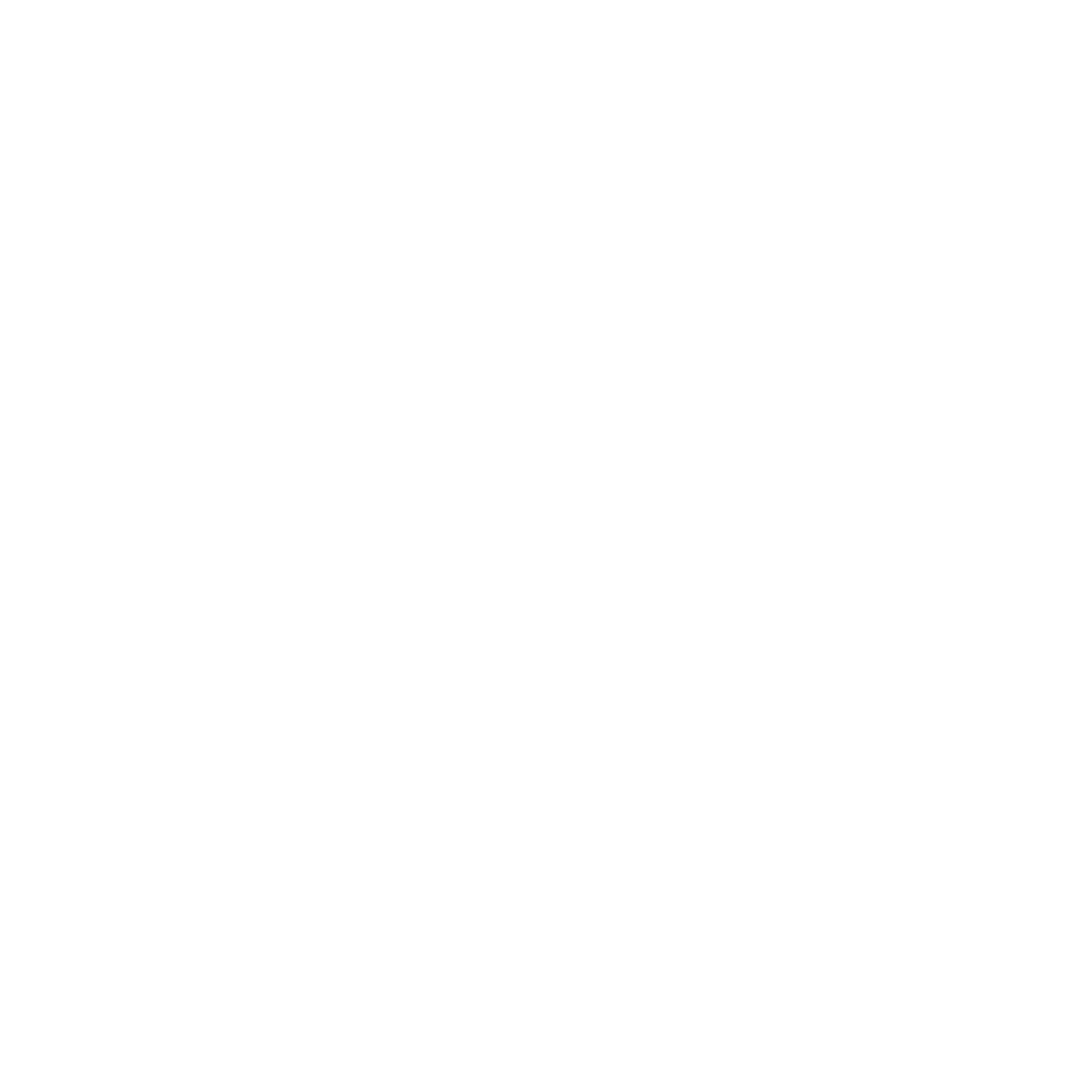
Also in the neuroscience category:
Sexually dimorphic role of diet and stress on behavior, energy metabolism, and the ventromedial hypothalamus
Jimeng Li

Enhancer-driven cell type comparison reveals similarities between the mammalian and bird pallium
Rodrigo Senovilla-Ganzo

Autism gene variants disrupt enteric neuron migration and cause gastrointestinal dysmotility
Rachel Mckeown

preListscell biology category:
in theBSCB-Biochemical Society 2024 Cell Migration meeting
This preList features preprints that were discussed and presented during the BSCB-Biochemical Society 2024 Cell Migration meeting in Birmingham, UK in April 2024. Kindly put together by Sara Morais da Silva, Reviews Editor at Journal of Cell Science.
List by | Reinier Prosee |
‘In preprints’ from Development 2022-2023
A list of the preprints featured in Development's 'In preprints' articles between 2022-2023
List by | Alex Eve, Katherine Brown |
preLights peer support – preprints of interest
This is a preprint repository to organise the preprints and preLights covered through the 'preLights peer support' initiative.
List by | preLights peer support |
The Society for Developmental Biology 82nd Annual Meeting
This preList is made up of the preprints discussed during the Society for Developmental Biology 82nd Annual Meeting that took place in Chicago in July 2023.
List by | Joyce Yu, Katherine Brown |
CSHL 87th Symposium: Stem Cells
Preprints mentioned by speakers at the #CSHLsymp23
List by | Alex Eve |
Journal of Cell Science meeting ‘Imaging Cell Dynamics’
This preList highlights the preprints discussed at the JCS meeting 'Imaging Cell Dynamics'. The meeting was held from 14 - 17 May 2023 in Lisbon, Portugal and was organised by Erika Holzbaur, Jennifer Lippincott-Schwartz, Rob Parton and Michael Way.
List by | Helen Zenner |
9th International Symposium on the Biology of Vertebrate Sex Determination
This preList contains preprints discussed during the 9th International Symposium on the Biology of Vertebrate Sex Determination. This conference was held in Kona, Hawaii from April 17th to 21st 2023.
List by | Martin Estermann |
Alumni picks – preLights 5th Birthday
This preList contains preprints that were picked and highlighted by preLights Alumni - an initiative that was set up to mark preLights 5th birthday. More entries will follow throughout February and March 2023.
List by | Sergio Menchero et al. |
CellBio 2022 – An ASCB/EMBO Meeting
This preLists features preprints that were discussed and presented during the CellBio 2022 meeting in Washington, DC in December 2022.
List by | Nadja Hümpfer et al. |
Fibroblasts
The advances in fibroblast biology preList explores the recent discoveries and preprints of the fibroblast world. Get ready to immerse yourself with this list created for fibroblasts aficionados and lovers, and beyond. Here, my goal is to include preprints of fibroblast biology, heterogeneity, fate, extracellular matrix, behavior, topography, single-cell atlases, spatial transcriptomics, and their matrix!
List by | Osvaldo Contreras |
EMBL Synthetic Morphogenesis: From Gene Circuits to Tissue Architecture (2021)
A list of preprints mentioned at the #EESmorphoG virtual meeting in 2021.
List by | Alex Eve |
FENS 2020
A collection of preprints presented during the virtual meeting of the Federation of European Neuroscience Societies (FENS) in 2020
List by | Ana Dorrego-Rivas |
Planar Cell Polarity – PCP
This preList contains preprints about the latest findings on Planar Cell Polarity (PCP) in various model organisms at the molecular, cellular and tissue levels.
List by | Ana Dorrego-Rivas |
BioMalPar XVI: Biology and Pathology of the Malaria Parasite
[under construction] Preprints presented at the (fully virtual) EMBL BioMalPar XVI, 17-18 May 2020 #emblmalaria
List by | Dey Lab, Samantha Seah |
1
Cell Polarity
Recent research from the field of cell polarity is summarized in this list of preprints. It comprises of studies focusing on various forms of cell polarity ranging from epithelial polarity, planar cell polarity to front-to-rear polarity.
List by | Yamini Ravichandran |
TAGC 2020
Preprints recently presented at the virtual Allied Genetics Conference, April 22-26, 2020. #TAGC20
List by | Maiko Kitaoka et al. |
3D Gastruloids
A curated list of preprints related to Gastruloids (in vitro models of early development obtained by 3D aggregation of embryonic cells). Updated until July 2021.
List by | Paul Gerald L. Sanchez and Stefano Vianello |
ECFG15 – Fungal biology
Preprints presented at 15th European Conference on Fungal Genetics 17-20 February 2020 Rome
List by | Hiral Shah |
ASCB EMBO Annual Meeting 2019
A collection of preprints presented at the 2019 ASCB EMBO Meeting in Washington, DC (December 7-11)
List by | Madhuja Samaddar et al. |
EMBL Seeing is Believing – Imaging the Molecular Processes of Life
Preprints discussed at the 2019 edition of Seeing is Believing, at EMBL Heidelberg from the 9th-12th October 2019
List by | Dey Lab |
Autophagy
Preprints on autophagy and lysosomal degradation and its role in neurodegeneration and disease. Includes molecular mechanisms, upstream signalling and regulation as well as studies on pharmaceutical interventions to upregulate the process.
List by | Sandra Malmgren Hill |
Lung Disease and Regeneration
This preprint list compiles highlights from the field of lung biology.
List by | Rob Hynds |
Cellular metabolism
A curated list of preprints related to cellular metabolism at Biorxiv by Pablo Ranea Robles from the Prelights community. Special interest on lipid metabolism, peroxisomes and mitochondria.
List by | Pablo Ranea Robles |
BSCB/BSDB Annual Meeting 2019
Preprints presented at the BSCB/BSDB Annual Meeting 2019
List by | Dey Lab |
MitoList
This list of preprints is focused on work expanding our knowledge on mitochondria in any organism, tissue or cell type, from the normal biology to the pathology.
List by | Sandra Franco Iborra |
Biophysical Society Annual Meeting 2019
Few of the preprints that were discussed in the recent BPS annual meeting at Baltimore, USA
List by | Joseph Jose Thottacherry |
ASCB/EMBO Annual Meeting 2018
This list relates to preprints that were discussed at the recent ASCB conference.
List by | Dey Lab, Amanda Haage |
Also in the neuroscience category:
‘In preprints’ from Development 2022-2023
A list of the preprints featured in Development's 'In preprints' articles between 2022-2023
List by | Alex Eve, Katherine Brown |
CSHL 87th Symposium: Stem Cells
Preprints mentioned by speakers at the #CSHLsymp23
List by | Alex Eve |
Journal of Cell Science meeting ‘Imaging Cell Dynamics’
This preList highlights the preprints discussed at the JCS meeting 'Imaging Cell Dynamics'. The meeting was held from 14 - 17 May 2023 in Lisbon, Portugal and was organised by Erika Holzbaur, Jennifer Lippincott-Schwartz, Rob Parton and Michael Way.
List by | Helen Zenner |
FENS 2020
A collection of preprints presented during the virtual meeting of the Federation of European Neuroscience Societies (FENS) in 2020
List by | Ana Dorrego-Rivas |
ASCB EMBO Annual Meeting 2019
A collection of preprints presented at the 2019 ASCB EMBO Meeting in Washington, DC (December 7-11)
List by | Madhuja Samaddar et al. |
SDB 78th Annual Meeting 2019
A curation of the preprints presented at the SDB meeting in Boston, July 26-30 2019. The preList will be updated throughout the duration of the meeting.
List by | Alex Eve |
Autophagy
Preprints on autophagy and lysosomal degradation and its role in neurodegeneration and disease. Includes molecular mechanisms, upstream signalling and regulation as well as studies on pharmaceutical interventions to upregulate the process.
List by | Sandra Malmgren Hill |
Young Embryologist Network Conference 2019
Preprints presented at the Young Embryologist Network 2019 conference, 13 May, The Francis Crick Institute, London
List by | Alex Eve |