ULK complex organization in autophagy by a C-shaped FIP200 N-terminal domain dimer
Posted on: 17 December 2019 , updated on: 18 December 2019
Preprint posted on 12 November 2019
Article now published in Journal of Cell Biology at http://dx.doi.org/10.1083/jcb.201911047
The FIP200 N-terminal domain orchestrates assembly of the autophagy-inducing ULK complex
Selected by Sandra Malmgren HillCategories: biochemistry, biophysics, cell biology, molecular biology
Context
The ULK complex is one of the most upstream and core complexes for regulating the cellular degradation and recycling process known as autophagy. Upon cellular cues, the ULK complex relocalizes to mark the sites of autophagy induction and recruits downstream effectors that eventually lead to the formation of a double membrane autophagosome, which encloses cargo and is sent to the lysosome for destruction1.
The human ULK complex consists of the serine/threonine kinase ULK1 (or its homologue ULK2) together with the scaffolding unit FIP200, ATG13, and ATG101. Upon activation and complex assembly, ULK1 autophosphorylates itself and the members of the ULK complex, as well as a range of substrates involved in different steps of autophagy regulation.
While a lot of functional studies have been carried out to describe the function of the ULK complex in relation to autophagy, information of the structure and assembly of the ULK complex itself is lacking. The authors of this pre-print focus on the FIP200 component and evaluate the possibility of this large protein acting as a scaffold for the assembly of the ULK complex. Using advanced technologies such as Hydrogen-deuterium exchange mass spectrometry, electron microscopy (EM) and Multiangle light scattering (MALS) to map the organization and stoichiometry of the complex, they show that two molecules of FIP200 dimerize at the N-terminal domain, forming a platform for the binding of ULK1, ATG13 and ATG101.
Main findings
FIP200 is the largest protein of the ULK complex, and consists of an N-terminal domain, a coiled-coiled domain and a C-terminal CLAW domain (Fig. 1A). The CLAW domain has been shown to be involved in binding to autophagy receptors, and the structure of this domain was recently solved2. The authors of this preprint determined that the N-terminal domain of FIP200 is essential for the interaction with the other components of the ULK complex. The authors purified the N-terminal domain alone (FIP200 NTD) to determine its structure and found that it forms a C-shaped dimer, with the C-terminal part of the domain at the dimer interface (Fig. 1B). The FIP200 dimer binds directly to ATG13, and ATG13 then interacts with ATG101 by heterodimerization via the HORMA domains resident in both proteins (Fig. 1A). In immunoprecipitation experiments, the authors showed that ATG13 and ATG101 could efficiently be pulled down by FIP200 NTD, whereas ULK1 could not, indicating that ULK1 recruitment to the complex requires the pre-existence of ATG13-ATG101.
The binding of ATG13-ATG101 stabilized the C-shaped structure of the FIP200 NTD dimer, and loss of binding had a destabilizing effect on the structure as well as on FIP200 protein levels. Further investigation using Hydrogen-Deuterium exchange mapped the region of interaction between FIP200 NTD and ATG13-ATG101 to the M6 region of FIP200 (aa443-450), and the middle region (MR; aa363-460) of ATG13 (Fig. 1A). Binding of the ULK1 EAT domain to ATG13 was mapped to the C-terminal region of ATG13, in agreement with previously published data3.
Figure 1. Interactions and assembly of the ULK complex. A) FIP200, ATG13, ATG101 and ULK1 visualized in linear form from amino (N) to carboxy terminal (C), with location of protein domains annotated by residue number. Interacting domains are denoted by connecting lines.B) Overview of the suggested ULK complex structure, with complex formation orchestrated by the FIP200 NTD, with the C-terminal of FIP200 (containing the adaptor binding CLAW domain) and the N-terminal of ULK1 (containing its kinase domain) protruding away from the interaction hub.
To localize the position of the interacting components in the structure, the authors tagged individual ULK components with a Maltose-binding-protein (MBP) tag, which is a two domain 40 kDa tag that is visible under electron microscopy. This analysis demonstrated that the ATG13 MR binds in the middle of the FIP200 NTD C shape, whereas the ULK1 EAT domain is located more towards the tip of the C-shape in the presence of ATG13 and ATG101 (Fig. 1B).
From these studies the authors could conclude that the ULK1 complex is asymmetric with a nonequal subunit stoichiometry of 2:1:1:1 FIP200:ATG13:ATG101:ULK1 (Fig. 1B), where the ULK complex assembly occurs at the FIP200 NTD dimer interface, with the C-terminal of FIP200 and the N-terminal of ULK1 projecting away from the complex assembly point.
Open questions
- Since there is no evidence of impaired autophagy when expressing ULK1 components with impaired in vitro binding, it remains to be determined how complex assembly is regulated in vivo. Are there additional adapters that could facilitate complex assembly, or are the proteins able to interact via other domains than those shown to be important for binding in vitro? As FIP200 was described to have a conserved TBK1 like domain, is it possible that TBK1 is involved in this process and that TBK1 could compensate to maintain adequate autophagy induction in the absence of a functional ULK complex?
- How is the complex assembly regulated in relation to autophagy induction? Do any of the known post-translational modifications on the ULK components affect FIP200 dimerization or complex assembly? ULK1 is known to autophosphorylate itself in the EAT domain to negatively regulate autophagy4 – could this regulation be further explained by a negative effect on complex assembly? Does FIP200 binding to autophagy adaptors influence the assembly of the complex?
- The C-shape described for the FIP200 NTD differs from its functional ortholog of Atg17 which is found as an S-shaped dimer, and the question remains how the C-shape influences function of the complex. The authors hypothesize that this shape could allow for binding to ATG9 vesicles or to the edge of a growing phagophore. It will be very interesting to see if further in vitro studies using liposomes of different sizes could shed a light on this matter.
- Is it possible to make any assumptions on the timing of the ULK complex assembly?
While immunoprecipitation data showed that there was no direct interaction between FIP200 and ULK1, indicating that ATG13 is acting as a linker between the two proteins, the authors later showed that FIP200 NTD could pull down ULK1 in the presence of ATG13ΔC, which can bind FIP200 but not ULK1. Is it possible that ULK1 binds an overlapping region on FIP200-ATG13 and that these two proteins need to interact before ULK1 is recruited to the complex? Or is it possible that ULK1 binds more than one region of ATG13 and that it can still interact with ATG13ΔC?
Why I chose this preprint
Autophagy is one of the major degradative pathways in the cell, essential for the proper maintenance and recycling of cellular material. Understanding its regulation and possible ways of manipulating this process is desirable in relation to many human ailments such as neurodegenerative diseases and cancer. This preprint highlights an important gap in our knowledge and provides information on one of the core players of autophagy induction. I find that this preprint, as well as other publications from the Hurley lab, effectively bridges the gap between structural and functional biology and provides a foundation for further studies on the FIP200 regulation of ULK complex.
References
1 Lin, M. G. & Hurley, J. H. Structure and function of the ULK1 complex in autophagy. Curr Opin Cell Biol 39, 61-68, doi:10.1016/j.ceb.2016.02.010 (2016).
2 Turco, E. et al. FIP200 Claw Domain Binding to p62 Promotes Autophagosome Formation at Ubiquitin Condensates. Mol Cell 74, 330-346 e311, doi:10.1016/j.molcel.2019.01.035 (2019).
3 Chan, E. Y., Longatti, A., McKnight, N. C. & Tooze, S. A. Kinase-inactivated ULK proteins inhibit autophagy via their conserved C-terminal domains using an Atg13-independent mechanism. Molecular and cellular biology 29, 157-171, doi:10.1128/MCB.01082-08 (2009).
4 Liu, C. C. et al. Cul3-KLHL20 Ubiquitin Ligase Governs the Turnover of ULK1 and VPS34 Complexes to Control Autophagy Termination. Mol Cell 61, 84-97, doi:10.1016/j.molcel.2015.11.001 (2016).
doi: https://doi.org/10.1242/prelights.15858
Read preprintSign up to customise the site to your preferences and to receive alerts
Register hereAlso in the biochemistry category:
Notch3 is a genetic modifier of NODAL signalling for patterning asymmetry during mouse heart looping
Bhaval Parmar
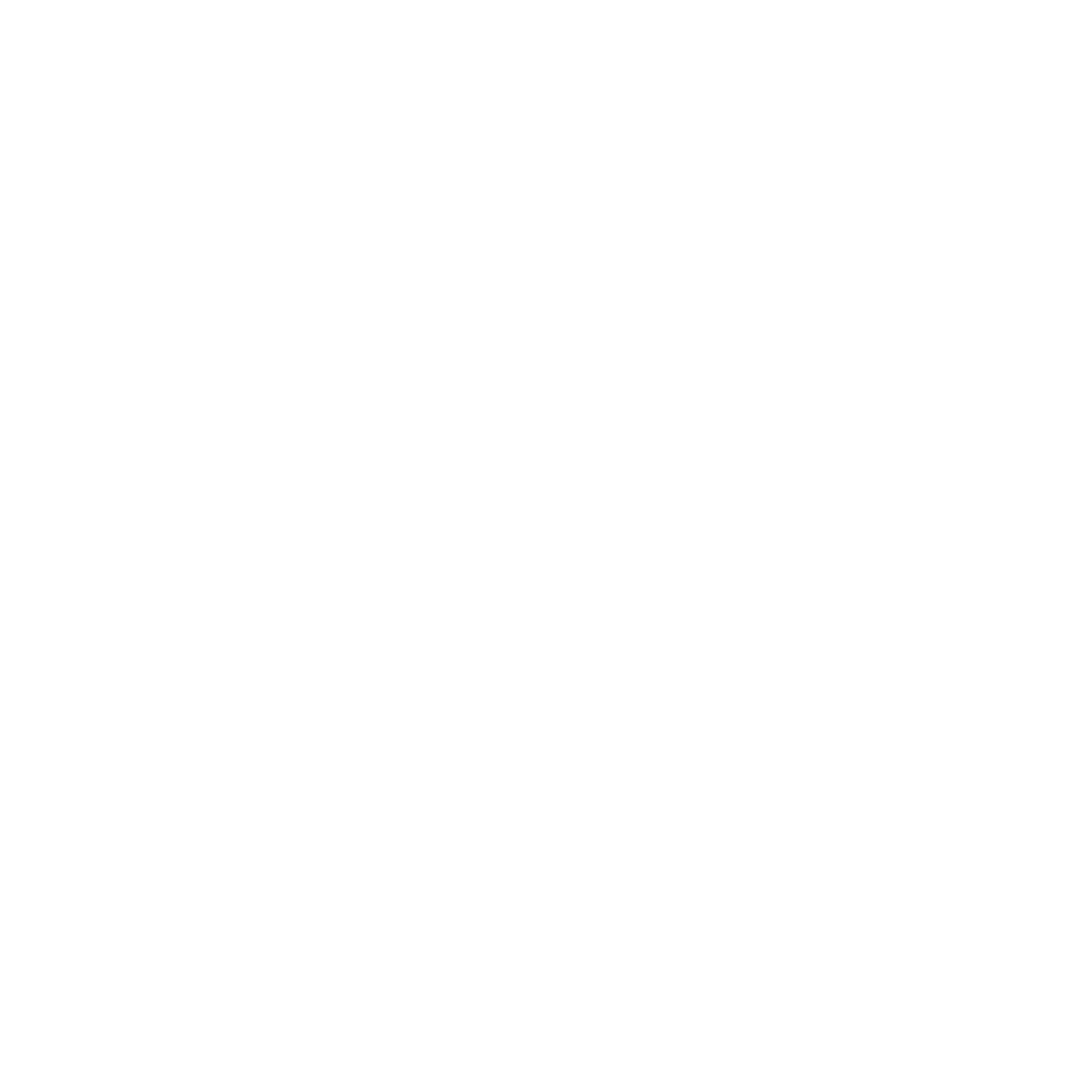
14-3-3 binding regulates Tau assembly and microtubule association
Barbora Knotkova et al.
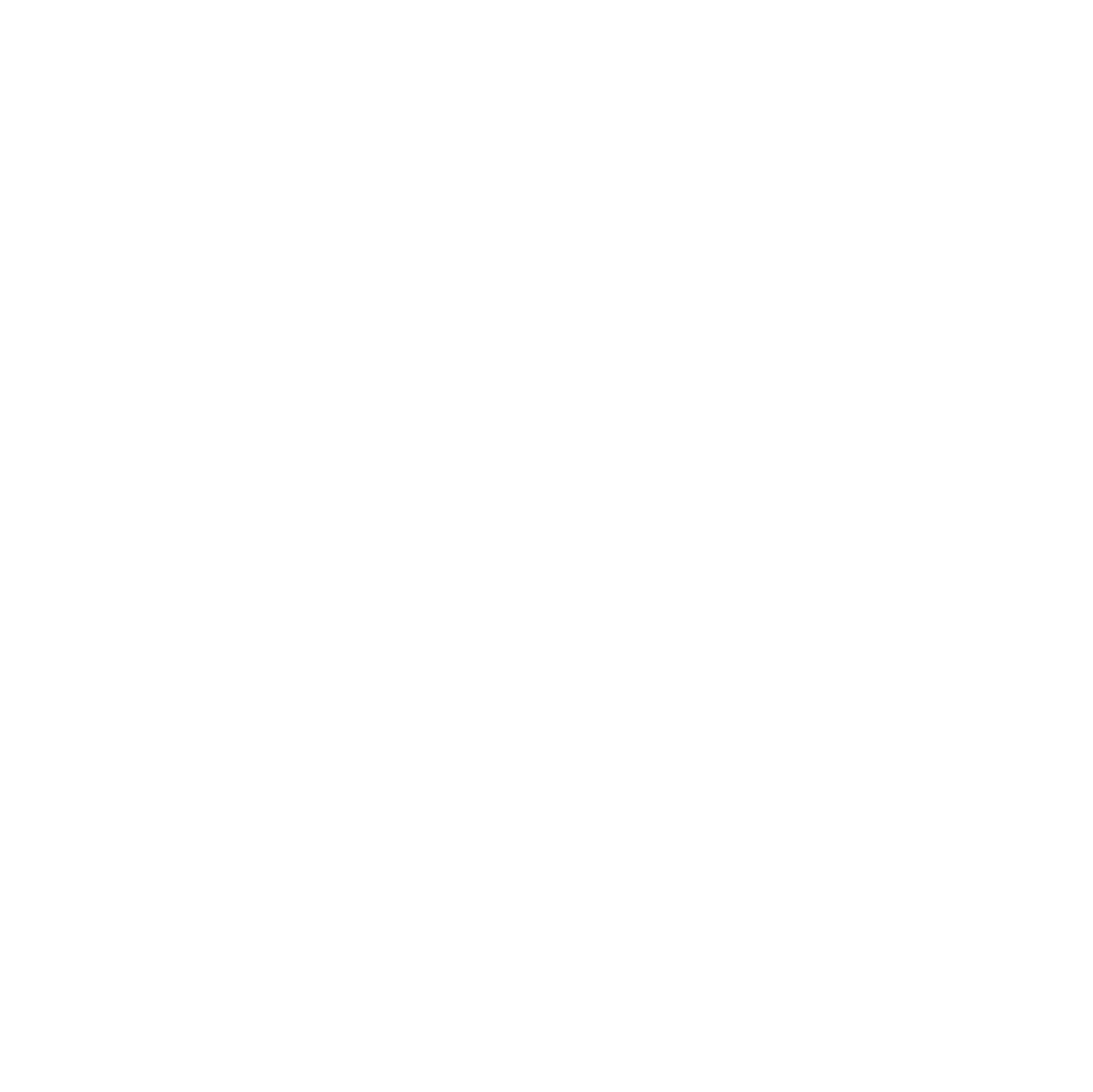
Structural basis of respiratory complexes adaptation to cold temperatures
Pamela Ornelas
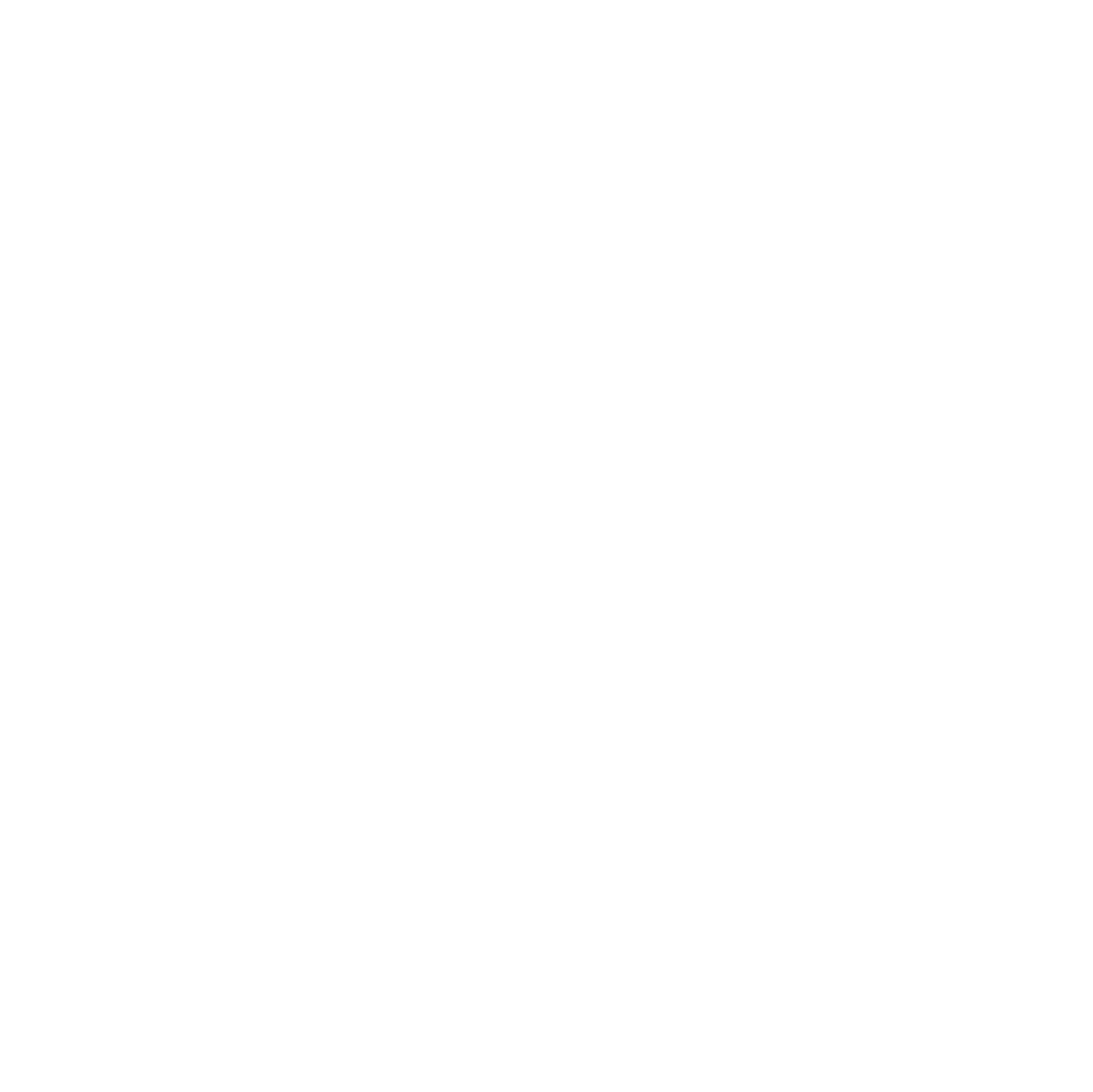
Also in the biophysics category:
Topology changes of the regenerating Hydra define actin nematic defects as mechanical organizers of morphogenesis
Rachel Mckeown
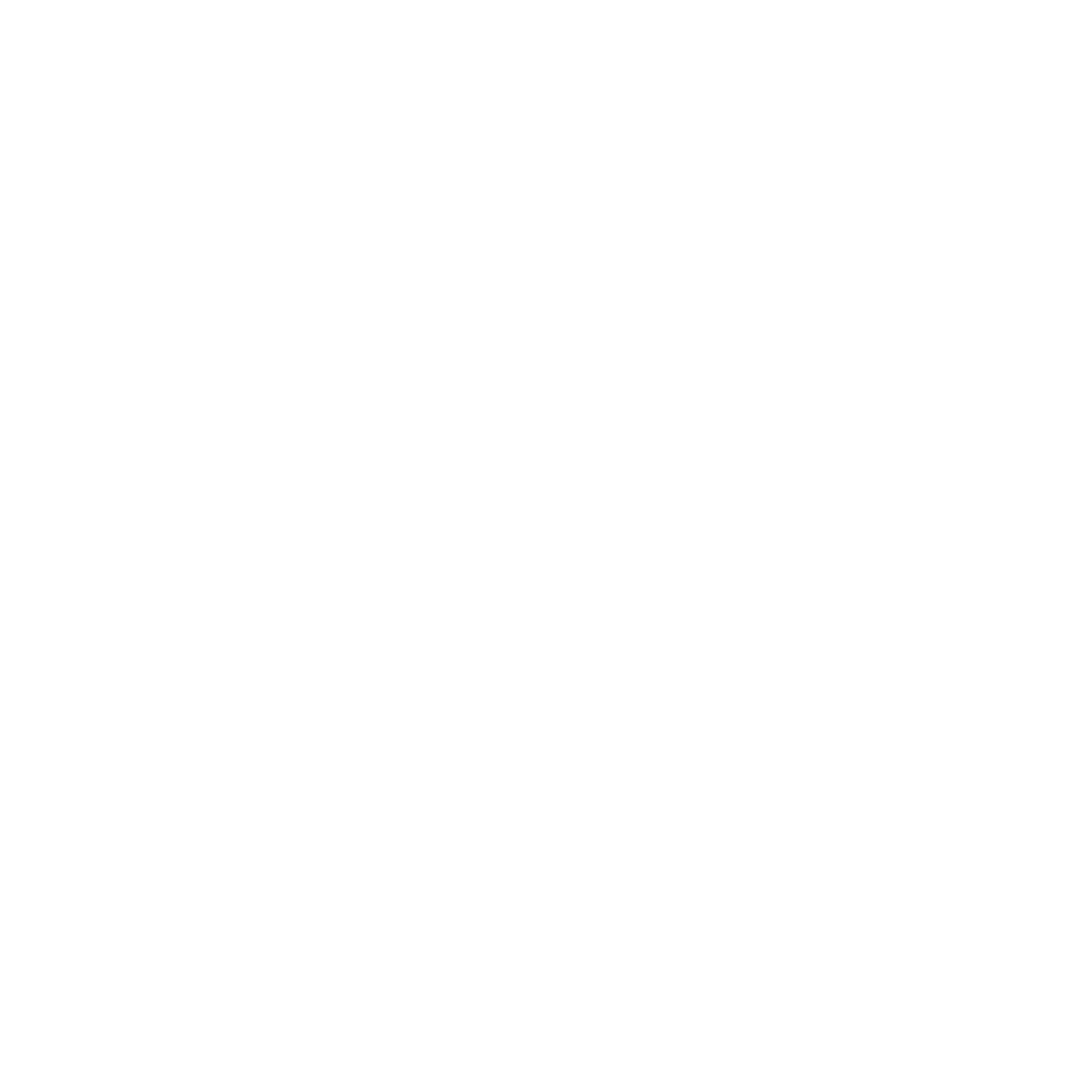
Actin polymerization drives lumen formation in a human epiblast model
Megane Rayer, Rivka Shapiro
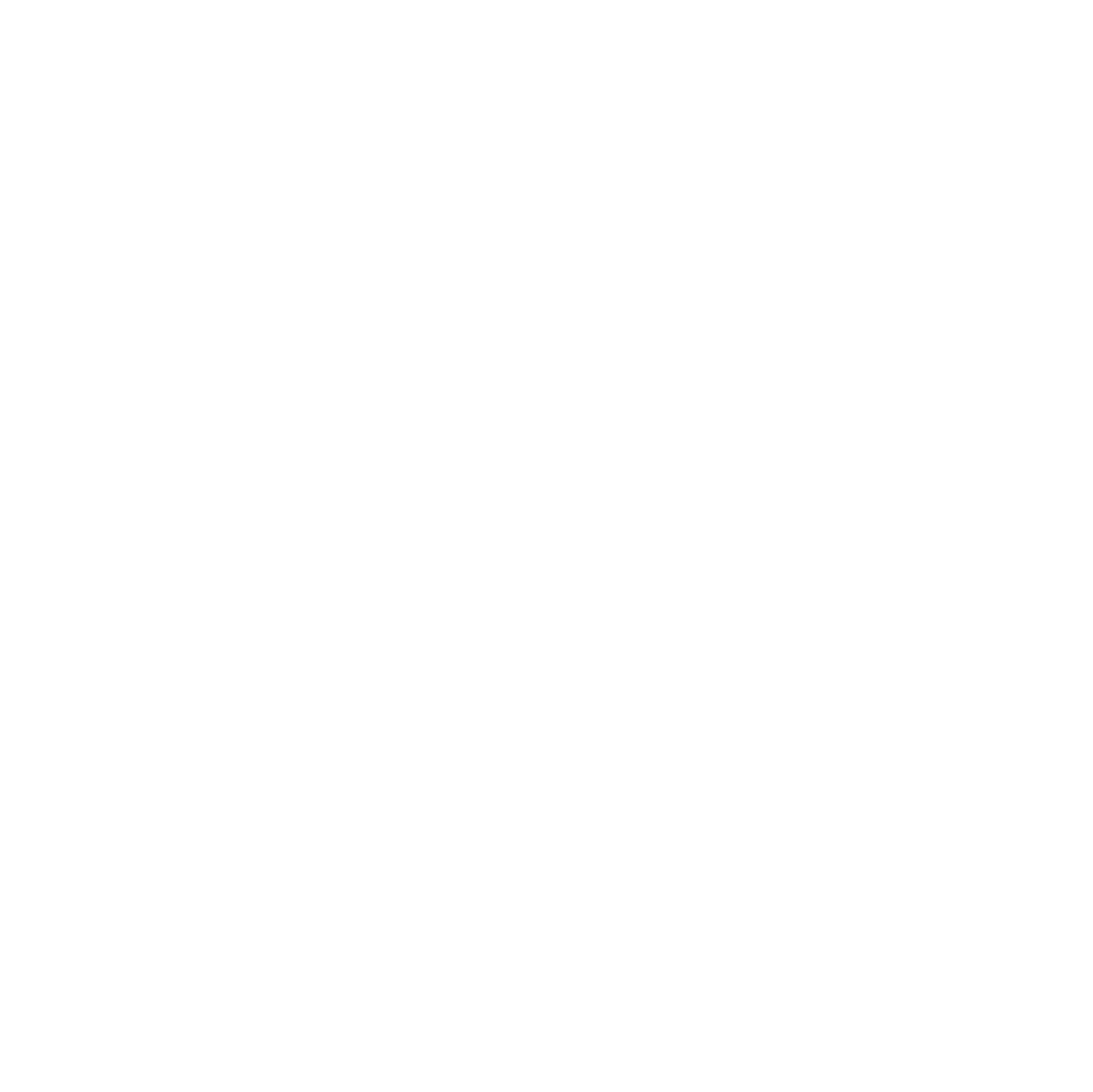
Learning a conserved mechanism for early neuroectoderm morphogenesis
Panagiotis Oikonomou
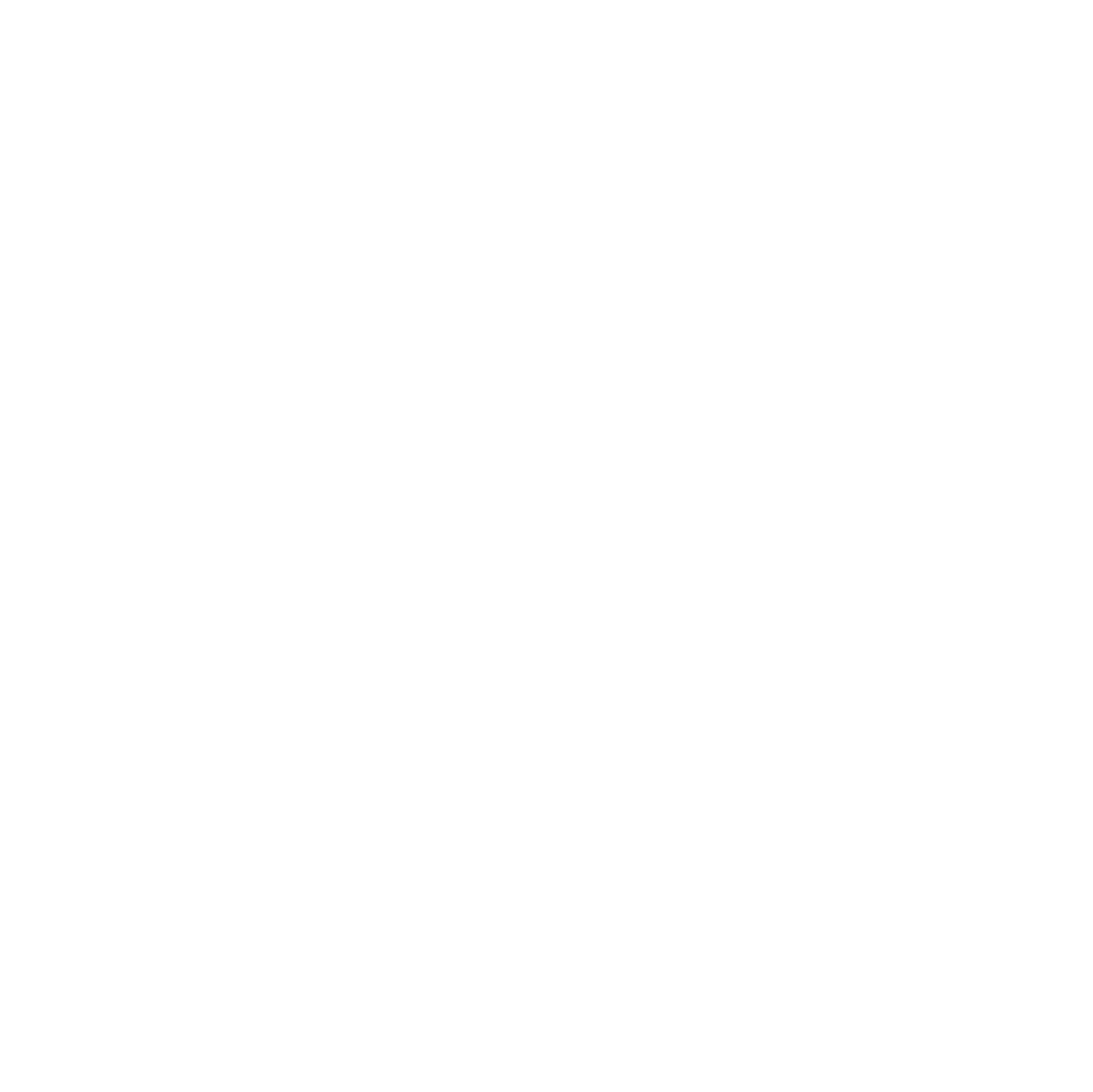
Also in the cell biology category:
Cell cycle-dependent mRNA localization in P-bodies
Mohammed JALLOH

Control of Inflammatory Response by Tissue Microenvironment
Roberto Amadio
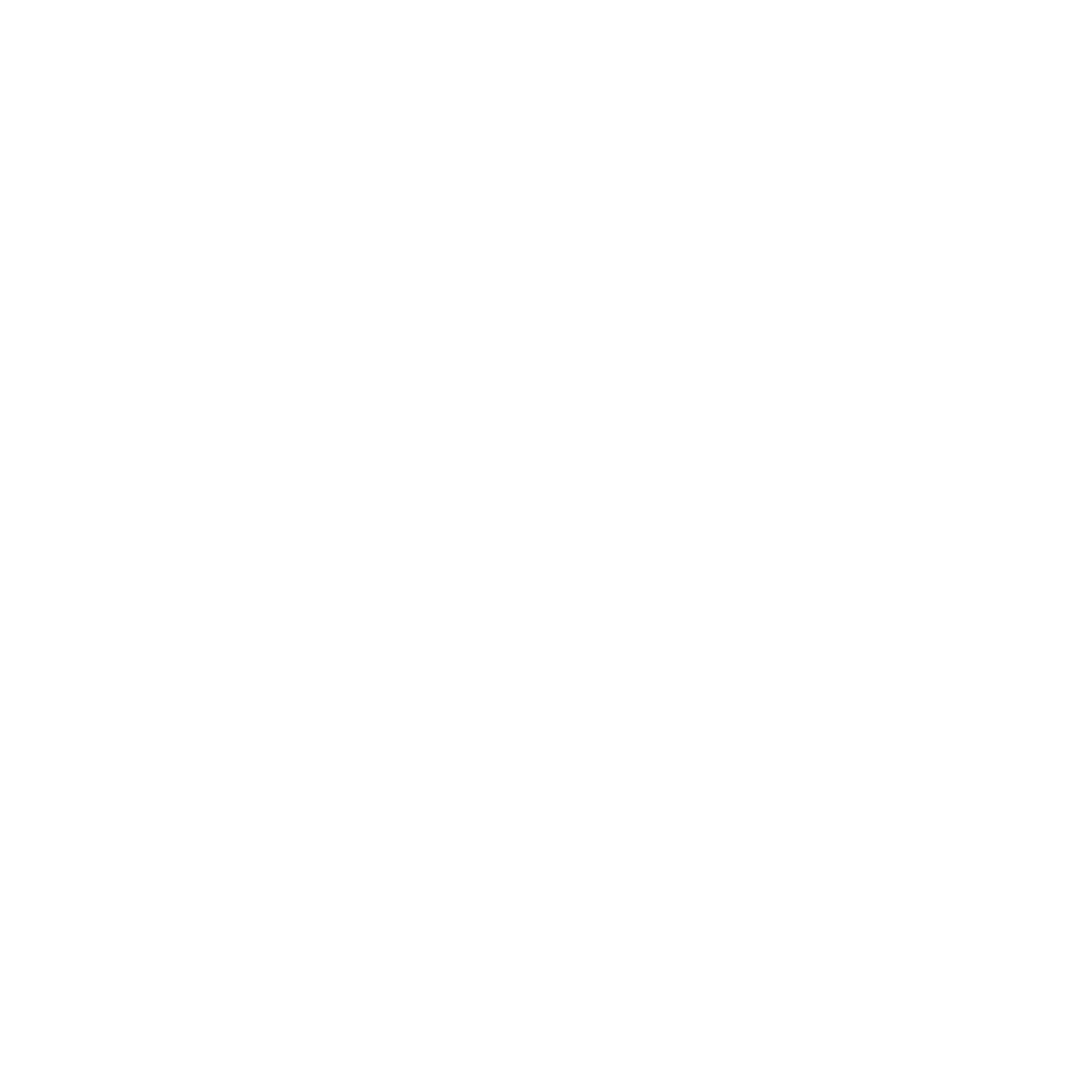
Notch3 is a genetic modifier of NODAL signalling for patterning asymmetry during mouse heart looping
Bhaval Parmar
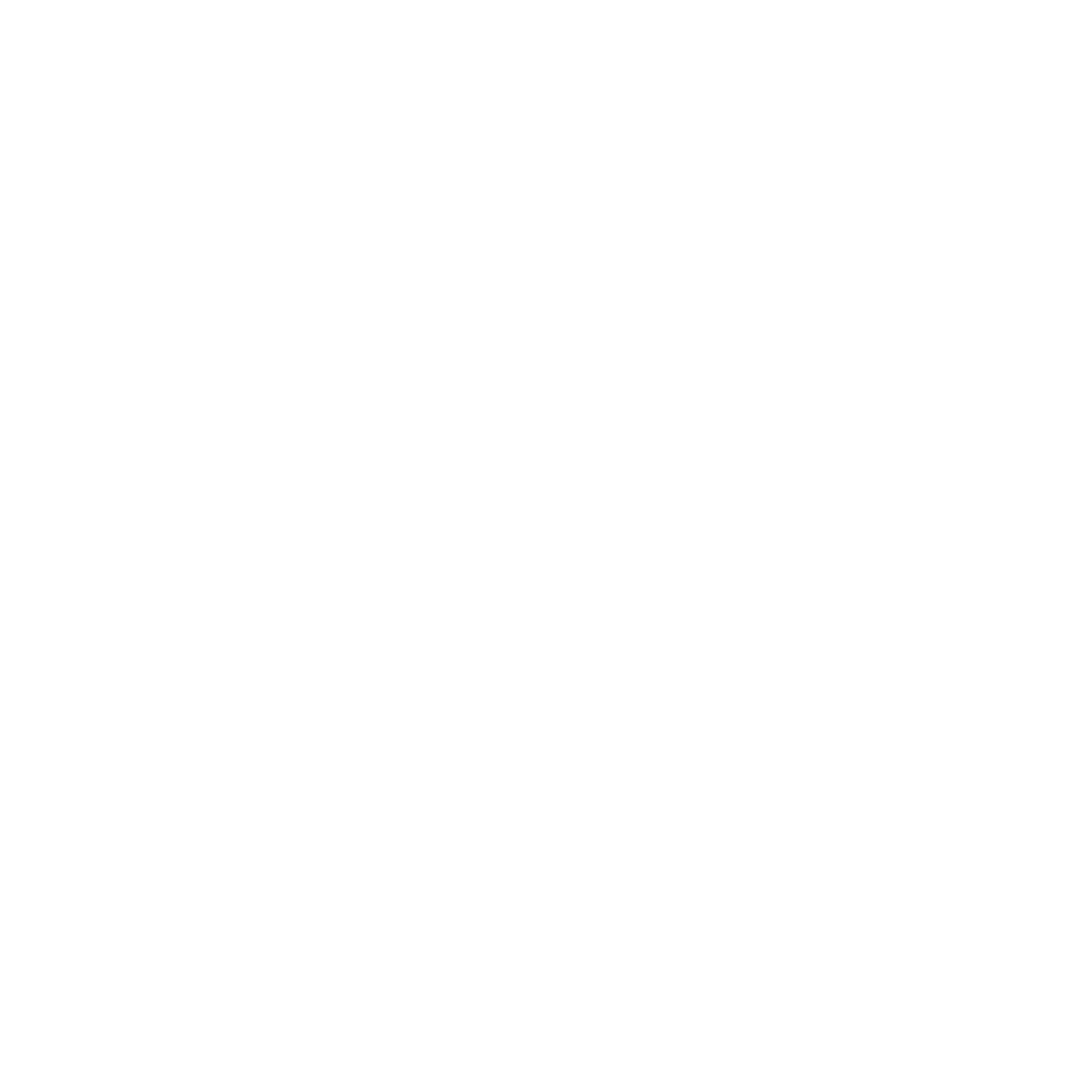
Also in the molecular biology category:
Cell cycle-dependent mRNA localization in P-bodies
Mohammed JALLOH

Fetal brain response to maternal inflammation requires microglia
Manuel Lessi

Clusters of lineage-specific genes are anchored by ZNF274 in repressive perinucleolar compartments
Silvia Carvalho

preListsbiochemistry category:
in theBSCB-Biochemical Society 2024 Cell Migration meeting
This preList features preprints that were discussed and presented during the BSCB-Biochemical Society 2024 Cell Migration meeting in Birmingham, UK in April 2024. Kindly put together by Sara Morais da Silva, Reviews Editor at Journal of Cell Science.
List by | Reinier Prosee |
Peer Review in Biomedical Sciences
Communication of scientific knowledge has changed dramatically in recent decades and the public perception of scientific discoveries depends on the peer review process of articles published in scientific journals. Preprints are key vehicles for the dissemination of scientific discoveries, but they are still not properly recognized by the scientific community since peer review is very limited. On the other hand, peer review is very heterogeneous and a fundamental aspect to improve it is to train young scientists on how to think critically and how to evaluate scientific knowledge in a professional way. Thus, this course aims to: i) train students on how to perform peer review of scientific manuscripts in a professional manner; ii) develop students' critical thinking; iii) contribute to the appreciation of preprints as important vehicles for the dissemination of scientific knowledge without restrictions; iv) contribute to the development of students' curricula, as their opinions will be published and indexed on the preLights platform. The evaluations will be based on qualitative analyses of the oral presentations of preprints in the field of biomedical sciences deposited in the bioRxiv server, of the critical reports written by the students, as well as of the participation of the students during the preprints discussions.
List by | Marcus Oliveira et al. |
CellBio 2022 – An ASCB/EMBO Meeting
This preLists features preprints that were discussed and presented during the CellBio 2022 meeting in Washington, DC in December 2022.
List by | Nadja Hümpfer et al. |
20th “Genetics Workshops in Hungary”, Szeged (25th, September)
In this annual conference, Hungarian geneticists, biochemists and biotechnologists presented their works. Link: http://group.szbk.u-szeged.hu/minikonf/archive/prg2021.pdf
List by | Nándor Lipták |
Fibroblasts
The advances in fibroblast biology preList explores the recent discoveries and preprints of the fibroblast world. Get ready to immerse yourself with this list created for fibroblasts aficionados and lovers, and beyond. Here, my goal is to include preprints of fibroblast biology, heterogeneity, fate, extracellular matrix, behavior, topography, single-cell atlases, spatial transcriptomics, and their matrix!
List by | Osvaldo Contreras |
ASCB EMBO Annual Meeting 2019
A collection of preprints presented at the 2019 ASCB EMBO Meeting in Washington, DC (December 7-11)
List by | Madhuja Samaddar et al. |
EMBL Seeing is Believing – Imaging the Molecular Processes of Life
Preprints discussed at the 2019 edition of Seeing is Believing, at EMBL Heidelberg from the 9th-12th October 2019
List by | Dey Lab |
Cellular metabolism
A curated list of preprints related to cellular metabolism at Biorxiv by Pablo Ranea Robles from the Prelights community. Special interest on lipid metabolism, peroxisomes and mitochondria.
List by | Pablo Ranea Robles |
MitoList
This list of preprints is focused on work expanding our knowledge on mitochondria in any organism, tissue or cell type, from the normal biology to the pathology.
List by | Sandra Franco Iborra |
Also in the biophysics category:
preLights peer support – preprints of interest
This is a preprint repository to organise the preprints and preLights covered through the 'preLights peer support' initiative.
List by | preLights peer support |
66th Biophysical Society Annual Meeting, 2022
Preprints presented at the 66th BPS Annual Meeting, Feb 19 - 23, 2022 (The below list is not exhaustive and the preprints are listed in no particular order.)
List by | Soni Mohapatra |
EMBL Synthetic Morphogenesis: From Gene Circuits to Tissue Architecture (2021)
A list of preprints mentioned at the #EESmorphoG virtual meeting in 2021.
List by | Alex Eve |
Biophysical Society Meeting 2020
Some preprints presented at the Biophysical Society Meeting 2020 in San Diego, USA.
List by | Tessa Sinnige |
ASCB EMBO Annual Meeting 2019
A collection of preprints presented at the 2019 ASCB EMBO Meeting in Washington, DC (December 7-11)
List by | Madhuja Samaddar et al. |
EMBL Seeing is Believing – Imaging the Molecular Processes of Life
Preprints discussed at the 2019 edition of Seeing is Believing, at EMBL Heidelberg from the 9th-12th October 2019
List by | Dey Lab |
Biomolecular NMR
Preprints related to the application and development of biomolecular NMR spectroscopy
List by | Reid Alderson |
Biophysical Society Annual Meeting 2019
Few of the preprints that were discussed in the recent BPS annual meeting at Baltimore, USA
List by | Joseph Jose Thottacherry |
Also in the cell biology category:
BSCB-Biochemical Society 2024 Cell Migration meeting
This preList features preprints that were discussed and presented during the BSCB-Biochemical Society 2024 Cell Migration meeting in Birmingham, UK in April 2024. Kindly put together by Sara Morais da Silva, Reviews Editor at Journal of Cell Science.
List by | Reinier Prosee |
‘In preprints’ from Development 2022-2023
A list of the preprints featured in Development's 'In preprints' articles between 2022-2023
List by | Alex Eve, Katherine Brown |
preLights peer support – preprints of interest
This is a preprint repository to organise the preprints and preLights covered through the 'preLights peer support' initiative.
List by | preLights peer support |
The Society for Developmental Biology 82nd Annual Meeting
This preList is made up of the preprints discussed during the Society for Developmental Biology 82nd Annual Meeting that took place in Chicago in July 2023.
List by | Joyce Yu, Katherine Brown |
CSHL 87th Symposium: Stem Cells
Preprints mentioned by speakers at the #CSHLsymp23
List by | Alex Eve |
Journal of Cell Science meeting ‘Imaging Cell Dynamics’
This preList highlights the preprints discussed at the JCS meeting 'Imaging Cell Dynamics'. The meeting was held from 14 - 17 May 2023 in Lisbon, Portugal and was organised by Erika Holzbaur, Jennifer Lippincott-Schwartz, Rob Parton and Michael Way.
List by | Helen Zenner |
9th International Symposium on the Biology of Vertebrate Sex Determination
This preList contains preprints discussed during the 9th International Symposium on the Biology of Vertebrate Sex Determination. This conference was held in Kona, Hawaii from April 17th to 21st 2023.
List by | Martin Estermann |
Alumni picks – preLights 5th Birthday
This preList contains preprints that were picked and highlighted by preLights Alumni - an initiative that was set up to mark preLights 5th birthday. More entries will follow throughout February and March 2023.
List by | Sergio Menchero et al. |
CellBio 2022 – An ASCB/EMBO Meeting
This preLists features preprints that were discussed and presented during the CellBio 2022 meeting in Washington, DC in December 2022.
List by | Nadja Hümpfer et al. |
Fibroblasts
The advances in fibroblast biology preList explores the recent discoveries and preprints of the fibroblast world. Get ready to immerse yourself with this list created for fibroblasts aficionados and lovers, and beyond. Here, my goal is to include preprints of fibroblast biology, heterogeneity, fate, extracellular matrix, behavior, topography, single-cell atlases, spatial transcriptomics, and their matrix!
List by | Osvaldo Contreras |
EMBL Synthetic Morphogenesis: From Gene Circuits to Tissue Architecture (2021)
A list of preprints mentioned at the #EESmorphoG virtual meeting in 2021.
List by | Alex Eve |
FENS 2020
A collection of preprints presented during the virtual meeting of the Federation of European Neuroscience Societies (FENS) in 2020
List by | Ana Dorrego-Rivas |
Planar Cell Polarity – PCP
This preList contains preprints about the latest findings on Planar Cell Polarity (PCP) in various model organisms at the molecular, cellular and tissue levels.
List by | Ana Dorrego-Rivas |
BioMalPar XVI: Biology and Pathology of the Malaria Parasite
[under construction] Preprints presented at the (fully virtual) EMBL BioMalPar XVI, 17-18 May 2020 #emblmalaria
List by | Dey Lab, Samantha Seah |
1
Cell Polarity
Recent research from the field of cell polarity is summarized in this list of preprints. It comprises of studies focusing on various forms of cell polarity ranging from epithelial polarity, planar cell polarity to front-to-rear polarity.
List by | Yamini Ravichandran |
TAGC 2020
Preprints recently presented at the virtual Allied Genetics Conference, April 22-26, 2020. #TAGC20
List by | Maiko Kitaoka et al. |
3D Gastruloids
A curated list of preprints related to Gastruloids (in vitro models of early development obtained by 3D aggregation of embryonic cells). Updated until July 2021.
List by | Paul Gerald L. Sanchez and Stefano Vianello |
ECFG15 – Fungal biology
Preprints presented at 15th European Conference on Fungal Genetics 17-20 February 2020 Rome
List by | Hiral Shah |
ASCB EMBO Annual Meeting 2019
A collection of preprints presented at the 2019 ASCB EMBO Meeting in Washington, DC (December 7-11)
List by | Madhuja Samaddar et al. |
EMBL Seeing is Believing – Imaging the Molecular Processes of Life
Preprints discussed at the 2019 edition of Seeing is Believing, at EMBL Heidelberg from the 9th-12th October 2019
List by | Dey Lab |
Autophagy
Preprints on autophagy and lysosomal degradation and its role in neurodegeneration and disease. Includes molecular mechanisms, upstream signalling and regulation as well as studies on pharmaceutical interventions to upregulate the process.
List by | Sandra Malmgren Hill |
Lung Disease and Regeneration
This preprint list compiles highlights from the field of lung biology.
List by | Rob Hynds |
Cellular metabolism
A curated list of preprints related to cellular metabolism at Biorxiv by Pablo Ranea Robles from the Prelights community. Special interest on lipid metabolism, peroxisomes and mitochondria.
List by | Pablo Ranea Robles |
BSCB/BSDB Annual Meeting 2019
Preprints presented at the BSCB/BSDB Annual Meeting 2019
List by | Dey Lab |
MitoList
This list of preprints is focused on work expanding our knowledge on mitochondria in any organism, tissue or cell type, from the normal biology to the pathology.
List by | Sandra Franco Iborra |
ASCB/EMBO Annual Meeting 2018
This list relates to preprints that were discussed at the recent ASCB conference.
List by | Dey Lab, Amanda Haage |
Also in the molecular biology category:
BSCB-Biochemical Society 2024 Cell Migration meeting
This preList features preprints that were discussed and presented during the BSCB-Biochemical Society 2024 Cell Migration meeting in Birmingham, UK in April 2024. Kindly put together by Sara Morais da Silva, Reviews Editor at Journal of Cell Science.
List by | Reinier Prosee |
‘In preprints’ from Development 2022-2023
A list of the preprints featured in Development's 'In preprints' articles between 2022-2023
List by | Alex Eve, Katherine Brown |
CSHL 87th Symposium: Stem Cells
Preprints mentioned by speakers at the #CSHLsymp23
List by | Alex Eve |
9th International Symposium on the Biology of Vertebrate Sex Determination
This preList contains preprints discussed during the 9th International Symposium on the Biology of Vertebrate Sex Determination. This conference was held in Kona, Hawaii from April 17th to 21st 2023.
List by | Martin Estermann |
Alumni picks – preLights 5th Birthday
This preList contains preprints that were picked and highlighted by preLights Alumni - an initiative that was set up to mark preLights 5th birthday. More entries will follow throughout February and March 2023.
List by | Sergio Menchero et al. |
CellBio 2022 – An ASCB/EMBO Meeting
This preLists features preprints that were discussed and presented during the CellBio 2022 meeting in Washington, DC in December 2022.
List by | Nadja Hümpfer et al. |
EMBL Synthetic Morphogenesis: From Gene Circuits to Tissue Architecture (2021)
A list of preprints mentioned at the #EESmorphoG virtual meeting in 2021.
List by | Alex Eve |
FENS 2020
A collection of preprints presented during the virtual meeting of the Federation of European Neuroscience Societies (FENS) in 2020
List by | Ana Dorrego-Rivas |
ECFG15 – Fungal biology
Preprints presented at 15th European Conference on Fungal Genetics 17-20 February 2020 Rome
List by | Hiral Shah |
ASCB EMBO Annual Meeting 2019
A collection of preprints presented at the 2019 ASCB EMBO Meeting in Washington, DC (December 7-11)
List by | Madhuja Samaddar et al. |
Lung Disease and Regeneration
This preprint list compiles highlights from the field of lung biology.
List by | Rob Hynds |
MitoList
This list of preprints is focused on work expanding our knowledge on mitochondria in any organism, tissue or cell type, from the normal biology to the pathology.
List by | Sandra Franco Iborra |