Mechanosensitive binding of p120-Catenin at cell junctions regulates E-Cadherin turnover and epithelial viscoelasticity
Posted on: 23 July 2018 , updated on: 24 July 2018
Preprint posted on 27 June 2018
Tissue viscoelasticity as a readout of tissue tension: mechanosensitive p120-Catenin regulates E-cadherin turnover at cellular junctions.
Selected by Ivana ViktorinováCategories: biophysics, cell biology, developmental biology
Background and summary
How tissues form remains a key open question in developmental biology. During animal development, tissues undergo dynamic reorganization that involves the coordinated behavior of cells including collective cell migration, cell rearrangements, cell shape/size changes and cell divisions/death. In the last decade, the field of developmental biology has been truly revolutionized by biophysical approaches that have revealed mechanical force (stress) in developing tissues. Therefore, to obtain a comprehensive picture of all developmental processes, it is essential to understand how genetic mechanisms and tissue mechanics work together.
Although most studies have focused on the generation of mechanical stress organized by biochemical signals (e.g. Heisenberg and Ballaïche, 2013), relatively little is known regarding how animal tissues actually respond to such stress (Chanet and Martin, 2014). A recent preprint by Iyer et al. provides a novel insights on the latter through the study of the developing Drosophila pupal wing. The authors show that the wing epithelium can be seen as a soft material with viscoelastic properties. This means that after mechanical stress has been applied to the wing tissue, the tissue does not reorganize instantly but instead with a delay (several hours in this case) and in irreversible manner. The authors also hypothesize and experimentally test whether mechanical stress influences the viscoelastic character/deformation of the wing epithelium at the molecular level. This preprint extends our knowledge about tissue deformation principles known from Drosophila embryonic germband extension (Rauzi et al. 2010).
Key findings
First, using circular and linear laser ablations, the authors find that the mechanical stress detected increases during hinge contraction in the developing wing at 20hAPF. This then gradually decreases and is followed six hours later by cell elongation along the proximal-distal (PD) axis of the pupal wing. Based on this, the authors conclude that the wing epithelium has viscoelastic properties. Importantly, their experiments convincingly show that mechanical stress is linked to cell elongation in the developing wing epithelium. Therefore, they propose that not only cell rearrangements, but also cell shape changes/cell elongations contribute to viscoelastic stress relaxation in this tissue.
Second, inspired by studies in cell cultures, the authors hypothesize whether applied stress can destabilize the core component of adherens junctions, E-cadherin. They wonder whether this mechanism could be the missing response to mechanical stress in the developing Drosophila wing. Using fluorescence recovery after photobleaching (FRAP) and genetic approaches, the authors find a portion of E-cadherin turnover that positively correlates with the timing of mechanical stress during hinge contraction in wild-type and also in mutant situations (dumpy mutant/RNAi, where mechanical stress is impaired). These findings lead the authors to suspect that mechanical stress may regulate E-cadherin turnover and specifically its destabilization from adherens junctions.
Third, using a combination of FRAP and laser ablations, the authors show that, unlike lateral diffusion, membrane trafficking significantly contributes to the stress-dependent portion of E-cadherin recovery. Using the temperature-sensitive Dynamin mutant shibirets, they also find that this membrane trafficking fraction of E-cadherin recovery derives from endocytosis.
So what controls E-cadherin destabilization from the cellular membrane during endocytosis? Inspired by other studies, the authors investigate the role of p120-Catenin, which is known to bind to the juxta-membrane domain of E-cadherin in other tissues/cells. The authors find that p120-Catenin is released from the membrane upon high mechanical stress and leads to E-cadherin destabilization from adherens junctions. This is lost in dumpy mutants, where p120 shows preferential junctional localization. Thus, the authors conclude that p120-Catenin is mechanosensitive.
Figure 7: Proposed model for regulation of tissue viscoelasticity by mechanosensitive binding of p120.
The authors wrap up the whole story by showing that in double mutants (dumpy and p120308) E-cadherin turnover is rescued to a comparable wild-type level, although the mechanical stress level does remain low. On the other hand, the single p120 mutant shows constantly elevated levels of mechanical stress after its peak during later wing development and it also reflected in early cell elongation (already 1h after mechanical stress normally increases). Therefore, the authors summarize that p120-Catenin is not only mechanosensitive and regulates E-cad turnover through endocytosis, but also defines the epithelial viscoelasticity of this tissue.
What I like about it
In this preprint, the authors combine non-invasive and elegant genetic (classical, temperature sensitive mutants, RNAi) experiments with sophisticated invasive physical approaches (circular/linear laser ablations and FRAP). As a researcher working with Drosophila myself, I am impressed by the combination of methods used in this preprint. I especially appreciate the hidden hard work behind laser ablations and FRAP experiments along with their subsequent analyses that are always time-consuming. Overall, the authors present their data very well and clearly lead the reader through their whole story. I also like that this work nicely shows how collaborations at the interface between biology and physics field can provide beautiful novel insights. An additional advantage is that the conclusions drawn here in this preprint open the possibility to apply this knowledge to other animal tissues.
Future directions and questions for the authors
The quality of this work is superb with strikingly beautiful clear figures and I have only a few questions for the authors:
- The authors propose that the analyzed tissue has viscoelastic properties. I wonder how the authors conclude that the 6h delay between mechanical stress and cell elongation/tissue deformation is an intermediate shift that is typical for viscoelastic materials? I can see mechanical stress peaks and declines, but has no oscillations to test a phase shift between stress and tissue response.
- The authors show a positive correlation between mechanical stress and E-cadherin internalization. Nevertheless, is it possible to perform a direct experiment (e.g. with optical tweezers) where mechanical stress is artificially/ectopically increased in the pupal wing to see whether it leads to an increase in E-cadherin internalization/p120-Catenin cytoplasmatic localization?
- On a similar note, cell proliferations/cell divisions are active until 22.5h pAPF in the investigated area of the pupal wing (Etournay et al. 2015). Have the authors thought that this mechanism could underlie E-cadherin turnover/its internalization to remodel adherens junctions?
- If mechanical stress leads to delayed cell elongation/cell rearrangements/tissue deformation through the p120-Catenin/E-cadherin complex and actomyosin regulation in the pupal wing, what could be the source of initial mechanical stress?
References
Chanet, S., and Martin, A.C. (2014). Mechanical force sensing in tissues. Prog Mol Biol Transl Sci 126, 317-352.
Etournay, R., Popovic, M., Merkel, M., Nandi, A., Blasse, C., Aigouy, B., Brandl, H., Myers, G., Salbreux, G., Julicher, F., et al. (2015). Interplay of cell dynamics and epithelial tension during morphogenesis of the Drosophila pupal wing. Elife 4, e07090.
Heisenberg, C.P., and Bellaiche, Y. (2013). Forces in tissue morphogenesis and patterning. Cell 153, 948-962.
Rauzi, M., Lenne, P.F., and Lecuit, T. (2010). Planar polarized actomyosin contractile flows control epithelial junction remodelling. Nature 468, 1110-1114.
Read preprintSign up to customise the site to your preferences and to receive alerts
Register hereAlso in the biophysics category:
Hyaluronic Acid and Emergent Tissue Mechanics Orchestrate Digit Tip Regeneration
Jonathan Townson
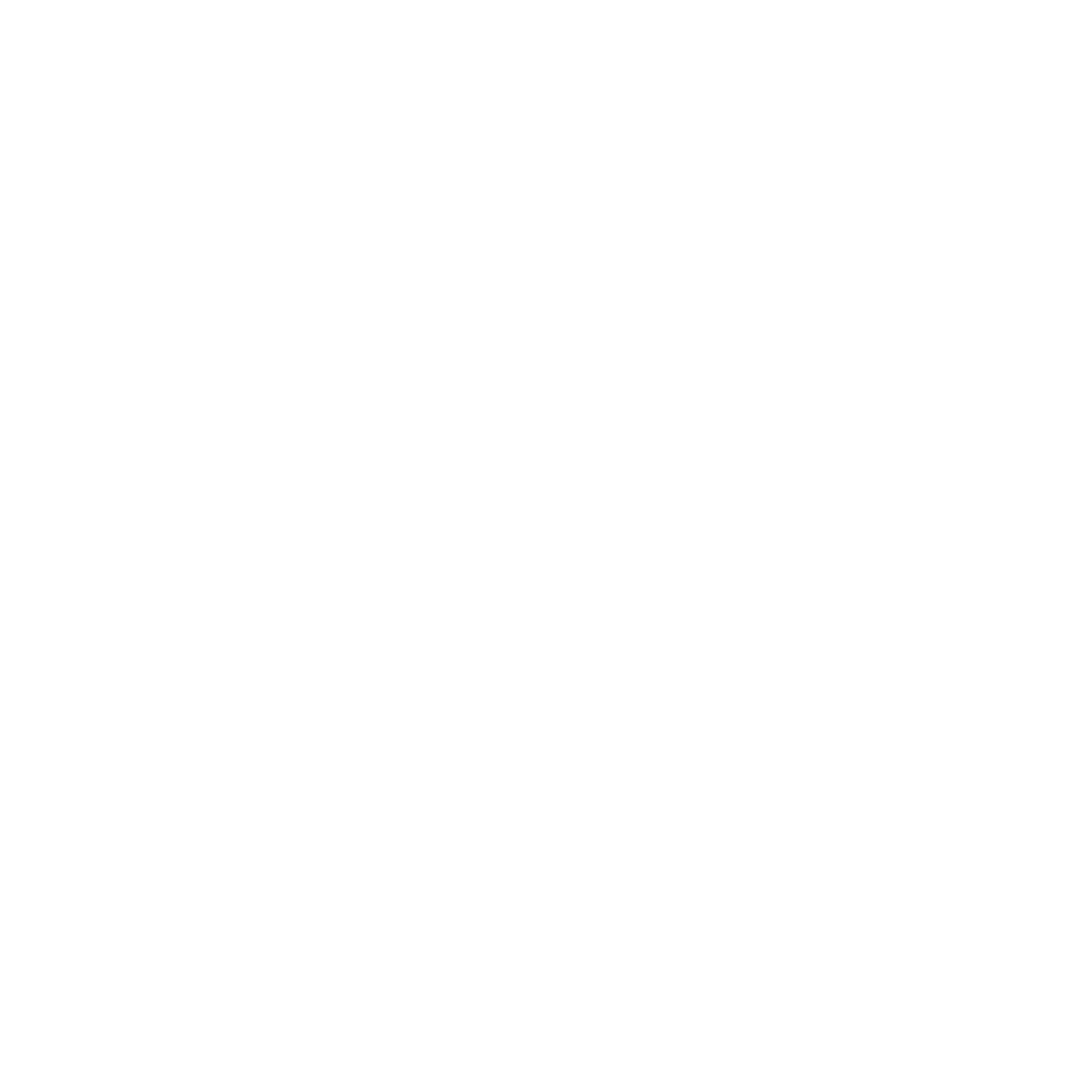
Target cell tension regulates macrophage trogocytosis
Felipe Del Valle Batalla
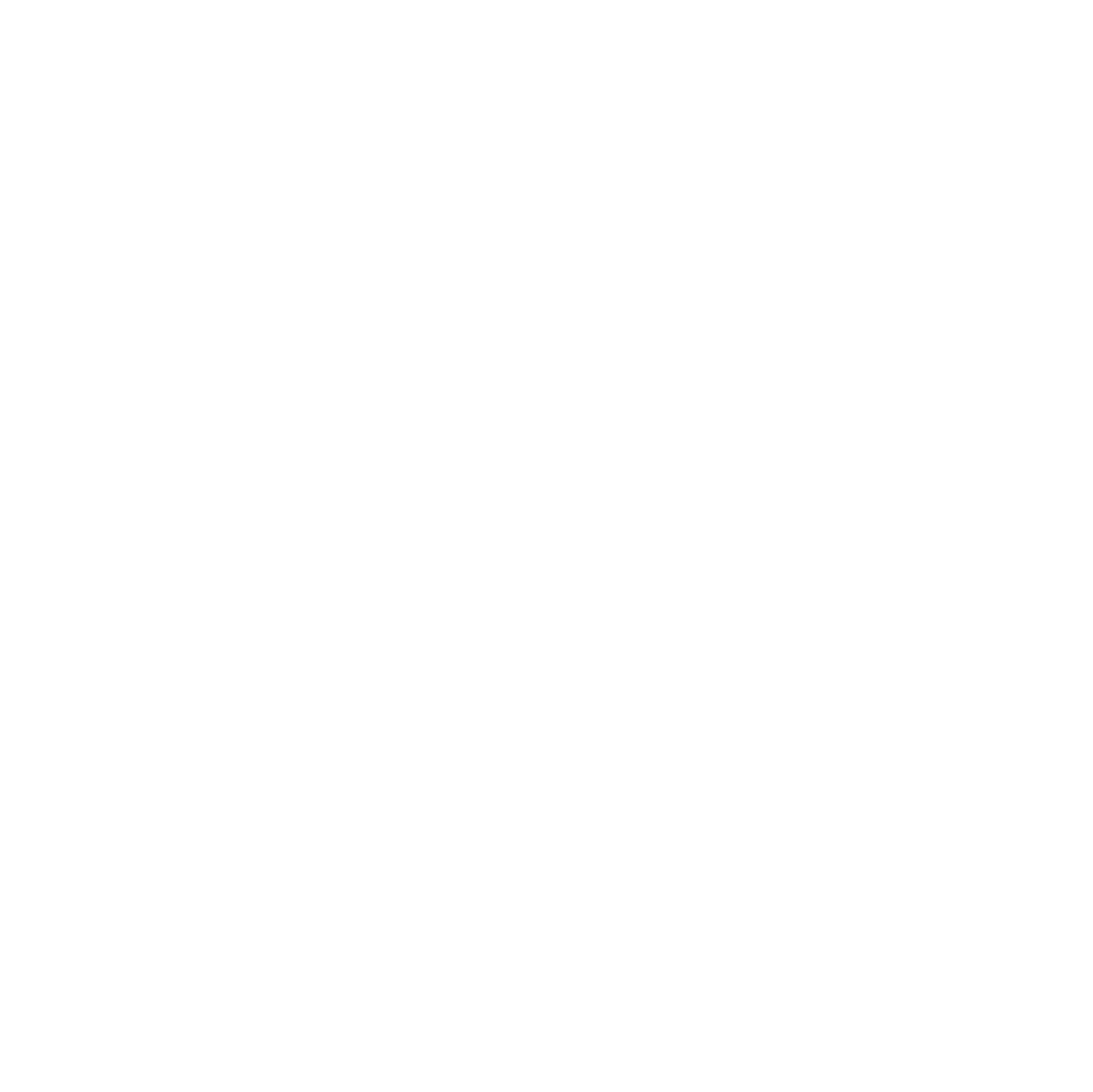
Restoring mechanophenotype reverts malignant properties of ECM-enriched vocal fold cancer
Teodora Piskova

Also in the cell biology category:
Deletion of PIEZO1 in adult cardiomyocytes accelerates cardiac aging and causes premature death
Theodora Stougiannou

Megakaryocytes assemble a three-dimensional cage of extracellular matrix that controls their maturation and anchoring to the vascular niche
Simon Cleary

Fis1 is required for the development of the dendritic mitochondrial network in pyramidal cortical neurons
Felipe Del Valle Batalla

Also in the developmental biology category:
Ectopic head regeneration after nervous system ablation in a sea anemone
Isabella Cisneros
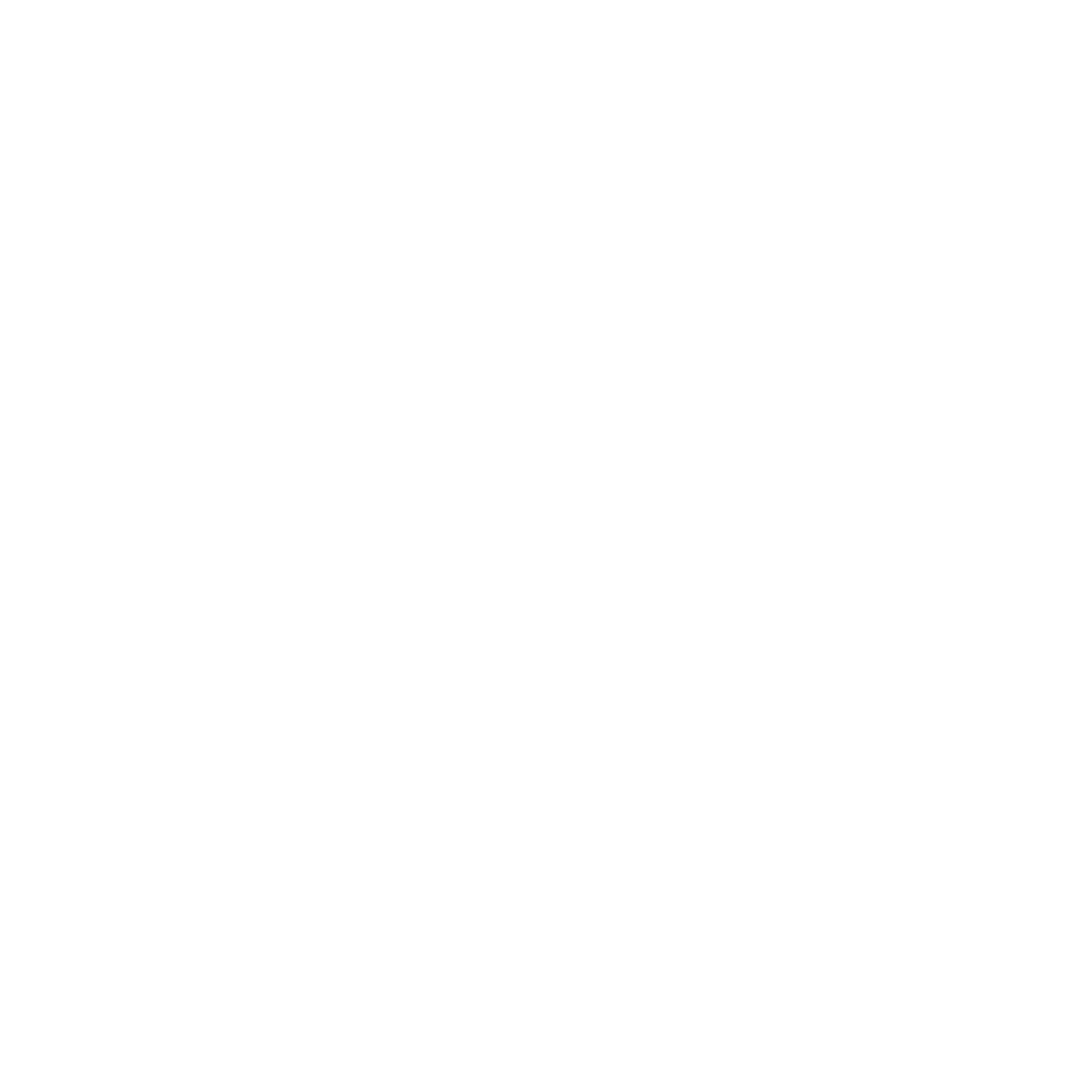
Hyaluronic Acid and Emergent Tissue Mechanics Orchestrate Digit Tip Regeneration
Jonathan Townson
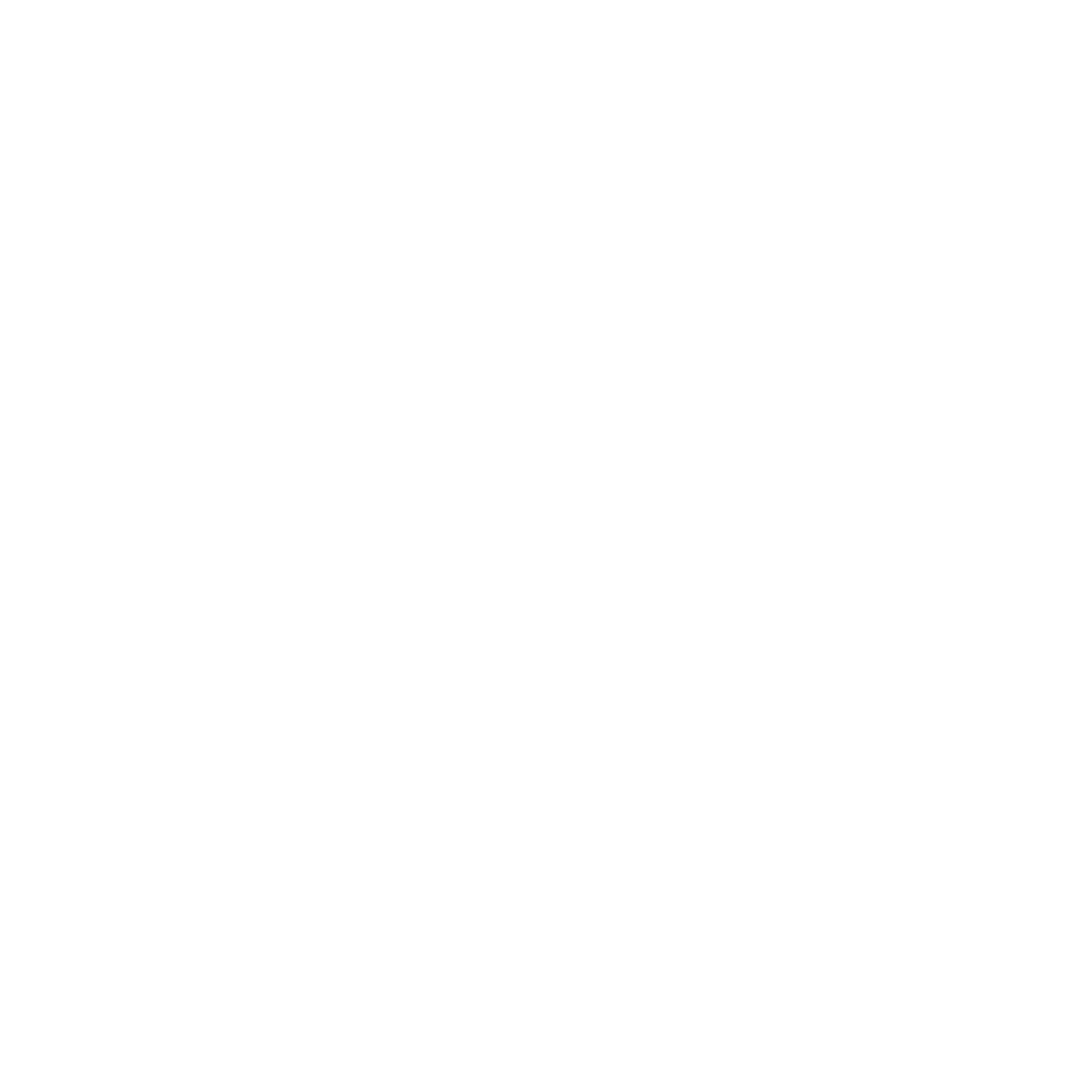
Visually-guided compensation of deafening-induced song deterioration
Maitri Manjunath

preLists in the biophysics category:
Biologists @ 100 conference preList
This preList aims to capture all preprints being discussed at the Biologists @100 conference in Liverpool, UK, either as part of the poster sessions or the (flash/short/full-length) talks.
List by | Jonathan Townson, Jonathan Townson |
February in preprints – the CellBio edition
A group of preLighters, with expertise in different areas of cell biology, have worked together to create this preprint reading lists for researchers with an interest in cell biology. This month, categories include: 1) biochemistry and cell metabolism 2) cell organelles and organisation 3) cell signalling, migration and mechanosensing
List by | Barbora Knotkova et al. |
preLights peer support – preprints of interest
This is a preprint repository to organise the preprints and preLights covered through the 'preLights peer support' initiative.
List by | preLights peer support |
66th Biophysical Society Annual Meeting, 2022
Preprints presented at the 66th BPS Annual Meeting, Feb 19 - 23, 2022 (The below list is not exhaustive and the preprints are listed in no particular order.)
List by | Soni Mohapatra |
EMBL Synthetic Morphogenesis: From Gene Circuits to Tissue Architecture (2021)
A list of preprints mentioned at the #EESmorphoG virtual meeting in 2021.
List by | Alex Eve |
Biophysical Society Meeting 2020
Some preprints presented at the Biophysical Society Meeting 2020 in San Diego, USA.
List by | Tessa Sinnige |
ASCB EMBO Annual Meeting 2019
A collection of preprints presented at the 2019 ASCB EMBO Meeting in Washington, DC (December 7-11)
List by | Madhuja Samaddar et al. |
EMBL Seeing is Believing – Imaging the Molecular Processes of Life
Preprints discussed at the 2019 edition of Seeing is Believing, at EMBL Heidelberg from the 9th-12th October 2019
List by | Dey Lab |
Biomolecular NMR
Preprints related to the application and development of biomolecular NMR spectroscopy
List by | Reid Alderson |
Biophysical Society Annual Meeting 2019
Few of the preprints that were discussed in the recent BPS annual meeting at Baltimore, USA
List by | Joseph Jose Thottacherry |
Also in the cell biology category:
Biologists @ 100 conference preList
This preList aims to capture all preprints being discussed at the Biologists @100 conference in Liverpool, UK, either as part of the poster sessions or the (flash/short/full-length) talks.
List by | Jonathan Townson, Jonathan Townson |
February in preprints – the CellBio edition
A group of preLighters, with expertise in different areas of cell biology, have worked together to create this preprint reading lists for researchers with an interest in cell biology. This month, categories include: 1) biochemistry and cell metabolism 2) cell organelles and organisation 3) cell signalling, migration and mechanosensing
List by | Barbora Knotkova et al. |
Community-driven preList – Immunology
In this community-driven preList, a group of preLighters, with expertise in different areas of immunology have worked together to create this preprint reading list.
List by | Felipe Del Valle Batalla et al. |
January in preprints – the CellBio edition
A group of preLighters, with expertise in different areas of cell biology, have worked together to create this preprint reading lists for researchers with an interest in cell biology. This month, categories include: 1) biochemistry/metabolism 2) cell migration 3) cell organelles and organisation 4) cell signalling and mechanosensing 5) genetics/gene expression
List by | Barbora Knotkova et al. |
December in preprints – the CellBio edition
A group of preLighters, with expertise in different areas of cell biology, have worked together to create this preprint reading lists for researchers with an interest in cell biology. This month, categories include: 1) cell cycle and division 2) cell migration and cytoskeleton 3) cell organelles and organisation 4) cell signalling and mechanosensing 5) genetics/gene expression
List by | Matthew Davies et al. |
November in preprints – the CellBio edition
This is the first community-driven preList! A group of preLighters, with expertise in different areas of cell biology, have worked together to create this preprint reading lists for researchers with an interest in cell biology. Categories include: 1) cancer cell biology 2) cell cycle and division 3) cell migration and cytoskeleton 4) cell organelles and organisation 5) cell signalling and mechanosensing 6) genetics/gene expression
List by | Felipe Del Valle Batalla et al. |
BSCB-Biochemical Society 2024 Cell Migration meeting
This preList features preprints that were discussed and presented during the BSCB-Biochemical Society 2024 Cell Migration meeting in Birmingham, UK in April 2024. Kindly put together by Sara Morais da Silva, Reviews Editor at Journal of Cell Science.
List by | Reinier Prosee |
‘In preprints’ from Development 2022-2023
A list of the preprints featured in Development's 'In preprints' articles between 2022-2023
List by | Alex Eve, Katherine Brown |
preLights peer support – preprints of interest
This is a preprint repository to organise the preprints and preLights covered through the 'preLights peer support' initiative.
List by | preLights peer support |
The Society for Developmental Biology 82nd Annual Meeting
This preList is made up of the preprints discussed during the Society for Developmental Biology 82nd Annual Meeting that took place in Chicago in July 2023.
List by | Joyce Yu, Katherine Brown |
CSHL 87th Symposium: Stem Cells
Preprints mentioned by speakers at the #CSHLsymp23
List by | Alex Eve |
Journal of Cell Science meeting ‘Imaging Cell Dynamics’
This preList highlights the preprints discussed at the JCS meeting 'Imaging Cell Dynamics'. The meeting was held from 14 - 17 May 2023 in Lisbon, Portugal and was organised by Erika Holzbaur, Jennifer Lippincott-Schwartz, Rob Parton and Michael Way.
List by | Helen Zenner |
9th International Symposium on the Biology of Vertebrate Sex Determination
This preList contains preprints discussed during the 9th International Symposium on the Biology of Vertebrate Sex Determination. This conference was held in Kona, Hawaii from April 17th to 21st 2023.
List by | Martin Estermann |
Alumni picks – preLights 5th Birthday
This preList contains preprints that were picked and highlighted by preLights Alumni - an initiative that was set up to mark preLights 5th birthday. More entries will follow throughout February and March 2023.
List by | Sergio Menchero et al. |
CellBio 2022 – An ASCB/EMBO Meeting
This preLists features preprints that were discussed and presented during the CellBio 2022 meeting in Washington, DC in December 2022.
List by | Nadja Hümpfer et al. |
Fibroblasts
The advances in fibroblast biology preList explores the recent discoveries and preprints of the fibroblast world. Get ready to immerse yourself with this list created for fibroblasts aficionados and lovers, and beyond. Here, my goal is to include preprints of fibroblast biology, heterogeneity, fate, extracellular matrix, behavior, topography, single-cell atlases, spatial transcriptomics, and their matrix!
List by | Osvaldo Contreras |
EMBL Synthetic Morphogenesis: From Gene Circuits to Tissue Architecture (2021)
A list of preprints mentioned at the #EESmorphoG virtual meeting in 2021.
List by | Alex Eve |
FENS 2020
A collection of preprints presented during the virtual meeting of the Federation of European Neuroscience Societies (FENS) in 2020
List by | Ana Dorrego-Rivas |
Planar Cell Polarity – PCP
This preList contains preprints about the latest findings on Planar Cell Polarity (PCP) in various model organisms at the molecular, cellular and tissue levels.
List by | Ana Dorrego-Rivas |
BioMalPar XVI: Biology and Pathology of the Malaria Parasite
[under construction] Preprints presented at the (fully virtual) EMBL BioMalPar XVI, 17-18 May 2020 #emblmalaria
List by | Dey Lab, Samantha Seah |
1
Cell Polarity
Recent research from the field of cell polarity is summarized in this list of preprints. It comprises of studies focusing on various forms of cell polarity ranging from epithelial polarity, planar cell polarity to front-to-rear polarity.
List by | Yamini Ravichandran |
TAGC 2020
Preprints recently presented at the virtual Allied Genetics Conference, April 22-26, 2020. #TAGC20
List by | Maiko Kitaoka et al. |
3D Gastruloids
A curated list of preprints related to Gastruloids (in vitro models of early development obtained by 3D aggregation of embryonic cells). Updated until July 2021.
List by | Paul Gerald L. Sanchez and Stefano Vianello |
ECFG15 – Fungal biology
Preprints presented at 15th European Conference on Fungal Genetics 17-20 February 2020 Rome
List by | Hiral Shah |
ASCB EMBO Annual Meeting 2019
A collection of preprints presented at the 2019 ASCB EMBO Meeting in Washington, DC (December 7-11)
List by | Madhuja Samaddar et al. |
EMBL Seeing is Believing – Imaging the Molecular Processes of Life
Preprints discussed at the 2019 edition of Seeing is Believing, at EMBL Heidelberg from the 9th-12th October 2019
List by | Dey Lab |
Autophagy
Preprints on autophagy and lysosomal degradation and its role in neurodegeneration and disease. Includes molecular mechanisms, upstream signalling and regulation as well as studies on pharmaceutical interventions to upregulate the process.
List by | Sandra Malmgren Hill |
Lung Disease and Regeneration
This preprint list compiles highlights from the field of lung biology.
List by | Rob Hynds |
Cellular metabolism
A curated list of preprints related to cellular metabolism at Biorxiv by Pablo Ranea Robles from the Prelights community. Special interest on lipid metabolism, peroxisomes and mitochondria.
List by | Pablo Ranea Robles |
BSCB/BSDB Annual Meeting 2019
Preprints presented at the BSCB/BSDB Annual Meeting 2019
List by | Dey Lab |
MitoList
This list of preprints is focused on work expanding our knowledge on mitochondria in any organism, tissue or cell type, from the normal biology to the pathology.
List by | Sandra Franco Iborra |
ASCB/EMBO Annual Meeting 2018
This list relates to preprints that were discussed at the recent ASCB conference.
List by | Dey Lab, Amanda Haage |
Also in the developmental biology category:
Biologists @ 100 conference preList
This preList aims to capture all preprints being discussed at the Biologists @100 conference in Liverpool, UK, either as part of the poster sessions or the (flash/short/full-length) talks.
List by | Jonathan Townson, Jonathan Townson |
BSDB/GenSoc Spring Meeting 2024
A list of preprints highlighted at the British Society for Developmental Biology and Genetics Society joint Spring meeting 2024 at Warwick, UK.
List by | Joyce Yu, Katherine Brown |
GfE/ DSDB meeting 2024
This preList highlights the preprints discussed at the 2024 joint German and Dutch developmental biology societies meeting that took place in March 2024 in Osnabrück, Germany.
List by | Joyce Yu |
‘In preprints’ from Development 2022-2023
A list of the preprints featured in Development's 'In preprints' articles between 2022-2023
List by | Alex Eve, Katherine Brown |
preLights peer support – preprints of interest
This is a preprint repository to organise the preprints and preLights covered through the 'preLights peer support' initiative.
List by | preLights peer support |
The Society for Developmental Biology 82nd Annual Meeting
This preList is made up of the preprints discussed during the Society for Developmental Biology 82nd Annual Meeting that took place in Chicago in July 2023.
List by | Joyce Yu, Katherine Brown |
CSHL 87th Symposium: Stem Cells
Preprints mentioned by speakers at the #CSHLsymp23
List by | Alex Eve |
Journal of Cell Science meeting ‘Imaging Cell Dynamics’
This preList highlights the preprints discussed at the JCS meeting 'Imaging Cell Dynamics'. The meeting was held from 14 - 17 May 2023 in Lisbon, Portugal and was organised by Erika Holzbaur, Jennifer Lippincott-Schwartz, Rob Parton and Michael Way.
List by | Helen Zenner |
9th International Symposium on the Biology of Vertebrate Sex Determination
This preList contains preprints discussed during the 9th International Symposium on the Biology of Vertebrate Sex Determination. This conference was held in Kona, Hawaii from April 17th to 21st 2023.
List by | Martin Estermann |
Alumni picks – preLights 5th Birthday
This preList contains preprints that were picked and highlighted by preLights Alumni - an initiative that was set up to mark preLights 5th birthday. More entries will follow throughout February and March 2023.
List by | Sergio Menchero et al. |
CellBio 2022 – An ASCB/EMBO Meeting
This preLists features preprints that were discussed and presented during the CellBio 2022 meeting in Washington, DC in December 2022.
List by | Nadja Hümpfer et al. |
2nd Conference of the Visegrád Group Society for Developmental Biology
Preprints from the 2nd Conference of the Visegrád Group Society for Developmental Biology (2-5 September, 2021, Szeged, Hungary)
List by | Nándor Lipták |
Fibroblasts
The advances in fibroblast biology preList explores the recent discoveries and preprints of the fibroblast world. Get ready to immerse yourself with this list created for fibroblasts aficionados and lovers, and beyond. Here, my goal is to include preprints of fibroblast biology, heterogeneity, fate, extracellular matrix, behavior, topography, single-cell atlases, spatial transcriptomics, and their matrix!
List by | Osvaldo Contreras |
EMBL Synthetic Morphogenesis: From Gene Circuits to Tissue Architecture (2021)
A list of preprints mentioned at the #EESmorphoG virtual meeting in 2021.
List by | Alex Eve |
EMBL Conference: From functional genomics to systems biology
Preprints presented at the virtual EMBL conference "from functional genomics and systems biology", 16-19 November 2020
List by | Jesus Victorino |
Single Cell Biology 2020
A list of preprints mentioned at the Wellcome Genome Campus Single Cell Biology 2020 meeting.
List by | Alex Eve |
Society for Developmental Biology 79th Annual Meeting
Preprints at SDB 2020
List by | Irepan Salvador-Martinez, Martin Estermann |
FENS 2020
A collection of preprints presented during the virtual meeting of the Federation of European Neuroscience Societies (FENS) in 2020
List by | Ana Dorrego-Rivas |
Planar Cell Polarity – PCP
This preList contains preprints about the latest findings on Planar Cell Polarity (PCP) in various model organisms at the molecular, cellular and tissue levels.
List by | Ana Dorrego-Rivas |
Cell Polarity
Recent research from the field of cell polarity is summarized in this list of preprints. It comprises of studies focusing on various forms of cell polarity ranging from epithelial polarity, planar cell polarity to front-to-rear polarity.
List by | Yamini Ravichandran |
TAGC 2020
Preprints recently presented at the virtual Allied Genetics Conference, April 22-26, 2020. #TAGC20
List by | Maiko Kitaoka et al. |
3D Gastruloids
A curated list of preprints related to Gastruloids (in vitro models of early development obtained by 3D aggregation of embryonic cells). Updated until July 2021.
List by | Paul Gerald L. Sanchez and Stefano Vianello |
ASCB EMBO Annual Meeting 2019
A collection of preprints presented at the 2019 ASCB EMBO Meeting in Washington, DC (December 7-11)
List by | Madhuja Samaddar et al. |
EDBC Alicante 2019
Preprints presented at the European Developmental Biology Congress (EDBC) in Alicante, October 23-26 2019.
List by | Sergio Menchero et al. |
EMBL Seeing is Believing – Imaging the Molecular Processes of Life
Preprints discussed at the 2019 edition of Seeing is Believing, at EMBL Heidelberg from the 9th-12th October 2019
List by | Dey Lab |
SDB 78th Annual Meeting 2019
A curation of the preprints presented at the SDB meeting in Boston, July 26-30 2019. The preList will be updated throughout the duration of the meeting.
List by | Alex Eve |
Lung Disease and Regeneration
This preprint list compiles highlights from the field of lung biology.
List by | Rob Hynds |
Young Embryologist Network Conference 2019
Preprints presented at the Young Embryologist Network 2019 conference, 13 May, The Francis Crick Institute, London
List by | Alex Eve |
Pattern formation during development
The aim of this preList is to integrate results about the mechanisms that govern patterning during development, from genes implicated in the processes to theoritical models of pattern formation in nature.
List by | Alexa Sadier |
BSCB/BSDB Annual Meeting 2019
Preprints presented at the BSCB/BSDB Annual Meeting 2019
List by | Dey Lab |
Zebrafish immunology
A compilation of cutting-edge research that uses the zebrafish as a model system to elucidate novel immunological mechanisms in health and disease.
List by | Shikha Nayar |